Modeling Failure Detection of Lift Doors Through Sensor Fusion
Apr 5, 2025
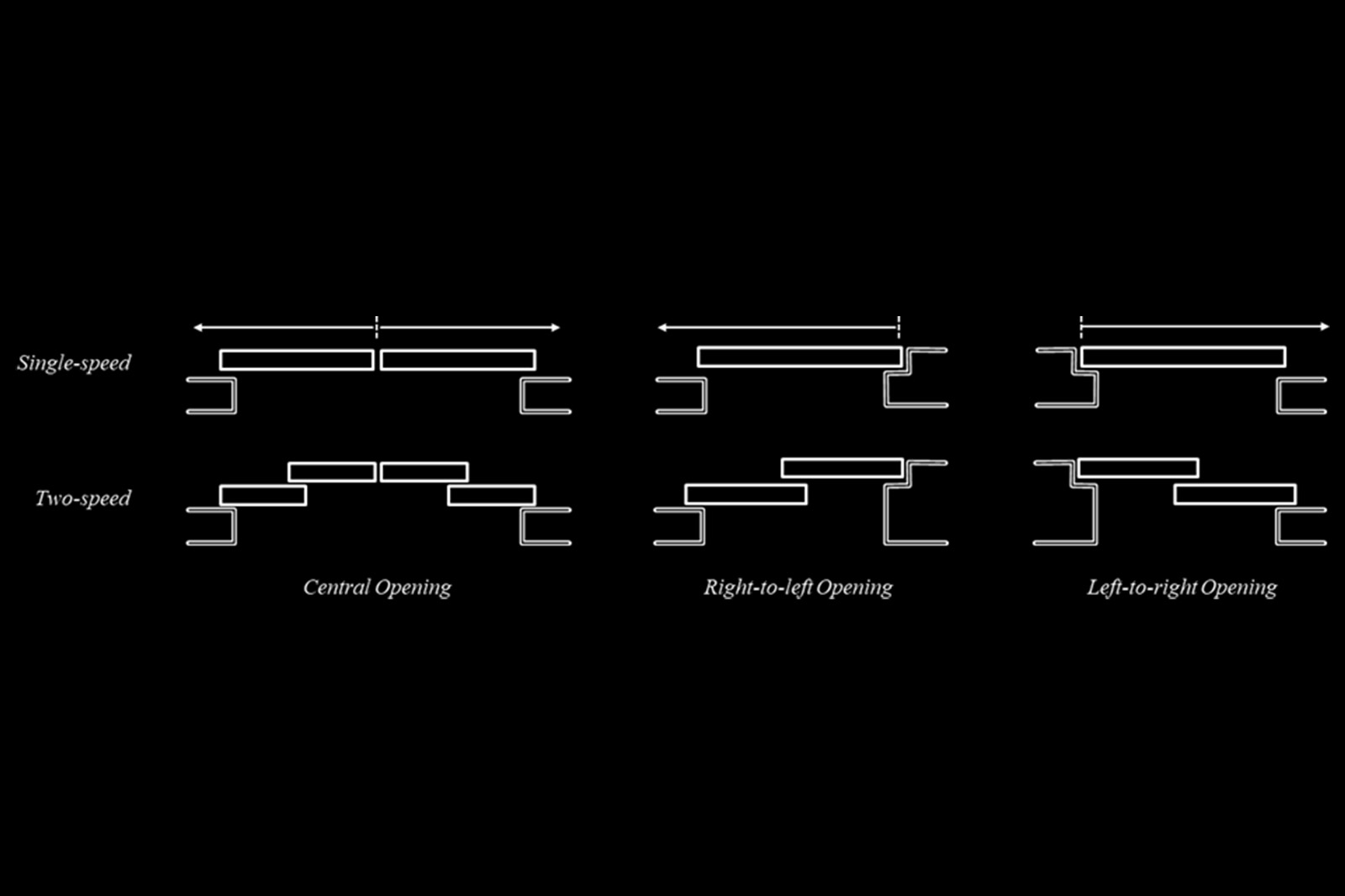
Emerging technologies improve the overall accuracy and reliability of detecting potential failures.
by Daan Smans
Keywords: Doors, Multiplier Effect, Sensor Fusion, Machine Learning, Internet of Things (IoT)
Abstract
Although steel and concrete make it possible to build ever higher, and glass and environmental control systems are making tall buildings liveable, it is the lifts that make such buildings actually usable. Unsurprisingly, lifts are increasingly forming the core of buildings.
A lift’s perceived value is largely dependent on its actual performance, typically expressed in terms of “availability” or “uptime.” Compensating downtime by adding additional lifts to the core of a building is not a viable solution. The extended footprint of the lift shaft(s) reduces the available tenable floorspace of the building, thus decreasing the economic viability of the building. Hence, downtime needs to be mitigated differently.
When examining the anatomy of the lift as a system, it becomes clear that the biggest variable of lift design is the number of doors needed. With a ratio of ≥1 per floor, the door system is the effort and material multiplier. Door systems are also among the busiest parts of the lift, typically cycling open and close with each loaded ride if not more often. Subsequently, defective door systems typically account for the majority of unplanned lift downtime.
Emerging technologies such as sensor fusion combine data from a multitude of sensors — i.e., optical sensors, accelerometers, gyroscopes, temperature, humidity, barometric pressure sensors, etc. — which improve the overall accuracy and reliability of detecting potential failures. Among other things, this makes it possible to monitor the technical health state of each and every individual door on a per-floor basis. When aggregating this vast amount of data over time, machine learning (ML) makes it possible to identify where issues may occur and when these need to be addressed. The Internet of Things (IoT) serving as backbone infrastructure enables these technologies to be scalable in an increasingly economic way.
1. Introduction
From a lift maintenance perspective, the doors are typically the key variable in assessing the overall maintenance effort required for proper equipment upkeep. Unlike other lift subsystems, the number of doors on a lift multiply with every floor of the building — especially when a lift is configured with both a front and a rear entrance to the cabin.
For the riding public to use a lift, doors need to cycle open and close at both the respective pick-up and destination floors for the purpose of letting passengers in or out. Therefore, doors are typically among the busiest parts of the lift. The industry standard is automatically operated sliding doors, as these increase both passenger flow efficiency as well as convenience. The moving parts required for this operation are subject to wear and tear that accumulates over time through ordinary use of the lift.
The wear and tear combined with the aforementioned multiplier effect — i.e. a ratio of ≥1 door(s) per floor — make the lift’s door system the key focus area of the overall lift maintenance effort. Moreover, the majority of lift faults overall occur in the lift’s door systems.[1] However: How does one determine the actual maintenance need on a per-door basis? By combining sensory data from sensors placed directly on and around the doors, data analytics on the basis of sensor fusion can aid maintenance personnel in making more informed decisions on what type of maintenance is needed where and when.
In support of said Conclusion (Section 5), this paper will cover the following areas:
- Anatomy of automatically operated sliding doors for lifts (foundational)
- Conventional approach to the maintenance of lift doors (relevant context)
- The concept of Sensor Fusion applied to lift doors (practical application)
2. Anatomy of Automatically Operated Sliding Doors for Lifts
The purpose of this section is to provide foundational knowledge on the workings of automatically operated sliding doors for lifts — today’s industry standard. This is done to make this paper more accessible as a standalone read for those not specialized in lift technology, but is by no means intended to be exhaustive. For further reading on lift door technology, the standard work Elevators 101 by Zack MaCain is recommended.
Over the 172-year history since Elisha Otis invented the safety hoist that made the modern lift as we know it today possible,[2] lift doors have featured various designs, often driven by the need for greater safety. Early lift door mechanisms consisted of simple hinged doors that opened and closed manually or with the assistance of mechanical systems.
The development of the modern lift door is primarily a 20th-century story.[3] As technology advanced, more sophisticated designs emerged. Today’s industry standard is automatically operated sliding doors. These types of doors open and close automatically through multiple panels that slide over each other in order to save space. Figure 1 provides a schematic overview of typical sliding door configurations.
Lift door mechanisms are engineered to ensure the safe and efficient loading and unloading of passengers and goods, as is applicable. The primary function of lift doors is opening and closing to facilitate entry and exit of the riding public in and out of the lift. For lift doors to operate automatically in a safe and convenient manner, sensors and control systems that detect passenger movement, respectively ensuring a timely and secure operation, are utilized.
Lifts use two different sets of doors, namely doors opening into the lift cabin (i.e., “cabin doors”) and doors opening into the lift shaft at each accessible landing of the building (i.e., “landing doors”). A fundamental requirement for lift door design is that doors are only allowed to operate if the floor of the lift cabin is — within accepted tolerance levels — aligned to a landing floor within the building. This is referred to as the landing zone of the lift cabin.
Therefore, there is only need for a single door operator located on the lift cabin. For the cabin doors to be able to open and close the landing doors safely and smoothly, the cabin doors use a clutch mechanism that unlocks the respective landing door, allowing the cabin door to pull them open or close in conjunction. The doors are operated by an electric motor, which is controlled by either a dedicated door control unit or otherwise by the lift’s main control system. There are two common types of door operator setups:
1) Harmonic door operator: a harmonic drive — i.e., an electric motor containing a mechanical speed-changing device that reduces the gear ratio of a rotary machine to increase torque — turns a wheel that is attached to a set of metal arms which pull or push the door panels to open or close.
2) Linear belt door operator: a linear drive — i.e., an electric motor that drives a horizontal toothed belt connected to the doors to cause them to open and close — that uses a belt to move the door panels to open or close.
The door panels are at their top side hung on tracks using a set of rollers and at their bottom side guided by gibs that slide through a door sill embedded in the cabin, respectively, in each of the landing floors. Typically, each door panel has two hanger rollers riding over the top of or otherwise inside the door tracks, and two upthrust rollers below the door tracks that prevent the hanger rollers from jumping out of the track. The gibs are typically static parts made of steel covered with plastic, felt or other type of durable yet comparatively soft material to ensure the smooth and noise-free sliding of the doors through the door sill.
There can be any number of reasons why lift doors would need to reopen unexpectedly. To mitigate the potential risk of bodily harm, damaging goods, respectively damaging the lift’s doors, automatically operated sliding doors need to have a reopening device (also known as a “safety edge”) to reopen the doors when obstructed by a passenger or an object. This can either be a mechanical-contact (typically “door bumpers”) or a non-contact design (typically “light curtains” or alternatively a “photo-eye” in older lift door models).
Both photo-eyes (single beam) and light curtains (multiple beams) operate based on infrared beams that keep the lift doors open as long as (any of) the beam(s) is (are) obstructed. This allows passengers to move freely in and out of the lift without the risk of any physical contact with the doors. The benefit of using a light curtain over a photo eye is that the former is able to detect almost the entire area of the doorway, whereas the latter only detects possible obstructions at a single point.
A well-designed light curtain incorporates methods of recognizing when the “beams” have been blocked for a considerable period. This feature — known as “nudging” — will alert and signal the person that they are blocking the doors. Nudging will cause the closing mechanism to operate at a lower speed and torque in order to gently close the doors but reopen them if needed.
Unlike light curtains, door bumpers (also known as “door buffers” or “mechanical safety edges”) will trigger the doors to retract only when they come into contact with a passenger or an object. While door bumpers are relatively safe, they can occasionally malfunction and subsequently not retract when colliding with a passenger. Consequentially, the majority of door bumpers have been replaced with light curtains that perform more reliably. Exceptions thereto can be found across several Asian countries — i.e., Japan, South Korea, etc. — where newer model door bumpers have light curtains embedded inside the door bumpers for added protection.
Landing doors are required to be closed and locked before the lift cabin is allowed to move. The exception to this is movement at the so called “leveling speed” — i.e., when a lift needs to realign the cabin floor with the respective landing floor — which is allowed with the doors open. The interlock (also known as “door lock”) is a safety mechanism that ensures that:
- The cabin cannot move unless all doors are securely closed;
- The landing doors are prevented from being opened unless the cabin is present;
- The lift doors are securely locked when the cabin is in motion.
During the 1970s and 1980s, there were several accidents involving lift passengers who manually pried open the cabin doors attempting to open the landing doors by manually releasing its interlock as means to self-evacuate from a lift stopped between floors.[4] To mitigate this safety risk, door restrictors were introduced to prevent the cabin doors from opening beyond a narrow gap when the cabin is not within its landing zone.
Notwithstanding all of the above, a manually operated mechanical override mechanism exists on every landing door, enabling authorized personnel — i.e., lift technicians — through use of a dedicated door unlocking key known as a “drop key” to have access to the lift shaft. This manual override can happen whether the lift cabin is located within its landing zone or not. Manual unlocking of the lift’s landing doors is typically done during lift maintenance or in the event of an emergency such as when passengers are entrapped.
For all of the above: Any moving part of the lift’s door system is susceptible to degradation over time through wear and tear due to normal use. However, depending on a number of factors —i.e., specific function, usage intensity, material used, climatic conditions, etc. — certain parts degrade faster than others. The following section will cover degradation of lift doors through wear and tear, as well as conventional practices for lift door maintenance.
3. Conventional Approach to the Maintenance of Lift Doors
Given the potentially severe consequences of unsafe lifts to the riding public, regulators across the globe have mandated regular preventive maintenance of lifts. The interval for preventive maintenance varies per market depending on the regulatory requirements laid out and enforced by the respective AHJ. For example, there are markets where the interval can largely be determined between the lift’s owner and the lift’s maintenance contractor (with at least one visit per year being mandatory) versus markets where the maintenance interval is regulatory prescribed.
The basic principles of conventional approaches to maintenance for any electro-mechanical equipment can be subdivided into two categories:
3) Preventive maintenance seeks to address equipment degradation caused by wear and tear through normal use on a regular basis. Wear and tear typically results in damage whereby the system is no longer operating in its ideal condition, but can still function satisfactorily.[5]
4) Corrective maintenance seeks to address equipment breakdowns whereby wear and tear has led to a fault in which the system can no longer operate satisfactorily (a change that produces an unacceptable reduction in quality).[5] Another cause of equipment breakdowns can be wrong use of the lift by passengers (both unintended/accidental as well as intended/vandalism).
The above applies to lift maintenance overall respectively and to lift doors specifically as well. In an effort to harmonize, steer and control maintenance activities, sets of widely accepted rules were codified. For example, the American Society of Mechanical Engineers (ASME) already included a section dedicated to maintenance when it published its first code in 1921.[6] Subsequently, companies specialized in lift maintenance have based their so-called Maintenance Control Programs (MCPs) upon such codes.
Essentially, preventative lift maintenance is a process of routine examination, lubrication, cleaning and adjustment of parts, components and/or subsystems for the purpose of ensuring that the lift’s performance complies with applicable code requirements. The MCPs steer this by prescribing the necessary work items to be performed, providing a place to record the completed work and containing the records of completion. It is commonly accepted that when adhering to these procedures diligently and consistently, preventive maintenance reduces the risk of unexpected lift downtime and simultaneously increases remaining useful lifetime as excessive degradation is prevented.
Specific to the lift’s door systems, Table 1 outlines what MCPs typically cover — though are not limited to — with respect to preventive and corrective maintenance activities.
Notwithstanding the many benefits that MCPs provide, the emergence of new technologies also highlighted the limitations of conventional MCPs. Most notably, conventional MCPs do not factor in key variables such as usage intensity over time, quality of the materials used, climatic conditions within the lift’s shaft and machine room, etc. Advancements in digital technologies are increasingly enabling the capabilities needed to factor in these dynamics. This, in turn, has led to the emergence of condition-based and predictive maintenance strategies. [7,8]
The following section will cover a brief history on the introduction of the first remote monitoring systems in the lift industry, their purposes, how these evolved over time and, ultimately, how this has led to the latest development on the basis of the sensor fusion concept.
Lift door equipment | Type |
Door operator, linkages and belt | I, A, R |
Gate switch and interlocks | I, A, R |
Cabin and landing door rollers | I, R |
Door speeds and times | I, A |
Door restrictors | I, A, R |
Door closers and relating devices | I, A, R |
Door tracks and hangars | I, C, L |
Door clutch and engaging mechanisms | I, A, L |
Door pressure | I, A |
Force closing, nudging | I, A |
Door gibs, site guards and astragals | I, A, R |
I = Inspect, L = Lubricate, T = Test, A = Adjust, C = Clean,
R = Repair or Replace
Table 1: Typical lift door equipment maintenance activities included in MCPs
4. The Concept of Sensor Fusion Applied to Lift Doors
Many of the world’s leading elevator companies developed and introduced their first versions of remote monitoring systems (RMS) nearly four decades ago. These first-generation systems were based predominantly on pre-processed data from the lift’s control system using rudimentary analytics and a few sensors. These systems were developed before the advent of the internet, thus typically storing the data on local databases. A brief trip down memory lane:
- Hitachi Elevator developed its first RMS system in 1987.
- Otis introduced “Remote Elevator Monitoring” (REM) in 1988, currently marketed as “OTIS – ONE.”
- Schindler introduced “Servitel” remote monitoring in the 1990s, currently marketed as “Schindler Ahead.”
- KONE introduced “KoneXion” in the 1990s, currently marketed as “KONE 24/7 Connected Services.”
- TK Elevator (TKE) first introduced “TeleService” remote monitoring in Europe, as well, in the 1990s, and later its first attempt at a next-generation product called “VISTA” in the U.S. in 2002. Its globally harmonized solution introduced in 2015 is marketed as “MAX.”
These first-generation systems brought innovation to the lift industry, making it an early adopter of remote monitoring technology. However, limitations in technology also meant that these original systems came with little workflow integration and, as such, inconsistent materialization of the benefits at best. The rapid rise and widespread adoption of the internet in the 1990s gave rise to the next generation of remote monitoring technology based on the IoT.
The term “Internet of Things” was first coined by British technology pioneer Kevin Ashton back in 1999.[9] Essentially, IoT refers to a network of physical items or “things” — within the context of this paper, “things” being lifts — that are embedded with sensors, software and other technologies with the purpose of connecting and sharing data with other devices and systems over the internet.
The aforementioned leading elevator companies once again became early adopters; IoT provided an infrastructure backbone that solved many of the prior technology limitations. For instance, Cloud technology provided accessibility from anywhere with any device, centralized data security, higher performance and availability, quick application development and unlimited storage capacity. Within the Cloud, ML technologies made it possible to process and adapt to vast amounts of data; by using statistical algorithms, ML could learn from data, generalize unseen data, and, in so doing, perform tasks without explicit instructions from humans.
However, the approach in terms of what type of input data to be used was not reassessed. The lift controller being the proverbial “brain and central nervous system” of the lift was generally deemed as capable of providing all the relevant data needed to bring lift maintenance to the next level. The general underlying assumption was that lift technicians already made extensive use of the lift controller’s diagnostic capabilities as the prime source of truth. The emphasis at the time was to harmonize workflows and centralize diagnostic toolsets. This meant that pre-processed data from lift control systems continued to remain prevalent.
Lift controller-centric IoT solutions soon proved to introduce their own constraints in terms of compatibility across different makes, models and generations of lift equipment, as well as limitations with data quality and usability. Compatibility limitations were the result of the wide variety of different hardware connectors used for interfacing with the lift controller, respectively how the software of the IoT edge device interacted with the lift controller. Many older generation lift controllers also did not provide the ability to interface at all and would require a modernization first. From a data perspective, in order to model failure detection, the ML needs to be able to ascertain how a lift functions within normal operating parameters, how these change in the lead-up toward a breakdown and, finally, what the breakdown actually looks like. The lift controller only provides error codes and status mode changes, which drastically limits the ML’s predictive capabilities.
Around the turn of the decade, the first IoT solutions using data directly from the sensor edge started to emerge in the industry. This is the time when the concept of Sensor Fusion came into play, as sensory inputs from multiple sensors needed to be processed simultaneously and interpreted wholistically. When properly synthesized, Sensor Fusion helps to reduce uncertainty in machine perception as each individual sensor comes with its own unique pros and cons. Using just one sensor to identify the surrounding environment is not sufficiently reliable, which translates to errors in the produced outcome. Conversely, Sensor Fusion algorithms process all inputs and subsequently produce outcomes with higher accuracy and reliability — even when individual measurements are not always sufficiently reliable.
The concept of Sensor Fusion thus resolves the limitations inherent to using the lift controller’s diagnostic data, i.e., error codes and status mode changes. Sensors can be applied universally, irrespective of make, model or equipment age. By using data taken directly from the sensor edge, data quality and usability limitations are negated by the ability to use the universal laws of physics. To concretize the Sensor Fusion concept, a concrete example of its practical application in relation to failure detection of lift doors will be provided.
Among others, some of the more common issues with sliding doors are:
- Gibs of the door panels being obstructed due to accumulation of debris in the door sill
- Misalignment of the electric contacts of the interlock, thus unable to close the safety circuit
- Electric contacts of the interlock getting sticky — i.e., small sparks known as “arcs” can corrode the contact-metal — leading to faulty circuits.
- Door rollers being worn out and therefore no longer able to properly open or close the door
The common denominator between the above four causes is that, typically, the lift doors need multiple attempts to fully close before the cabin can take off. This phenomenon is typically referred to as door cycling.
By using optical sensors, such as those of a light curtain, the opening and closing time can be derived from the light intensity of the beams being transmitted and received between the two individual light curtains. Light curtains are also capable of filtering out intentional door reversals — which could be counted as an above average door cycle when unable to validate — versus unintentional door reversals.
When adding an accelerometer to each individual light curtain, the vibration of the door panels can be observed; even though the light curtains are installed on the cabin doors, due to the mechanical clutch mechanism linking the cabin door and hall door together, the vibration of the hall doors are passed through into the cabin doors as well and, as such, observed.
By adding a barometric pressure sensor, the position of the cabin relative to the floors of the building can be determined. As a standalone sensor, there is increased risk of inaccuracy; therefore, an additional accelerometer should be placed on the cabin to measure acceleration and velocity to derive the distance traveled from its last known location.
When fusing all the aforementioned sensory inputs together, the door performance can be observed on a per-floor basis. The accuracy of the cabin positioning is further improved by the door vibration signature being a unique “fingerprint” for each floor respectively. Similarly, the differentiation per floor provides for the best comparable references to determine the individual lift’s ideal door performance parameters. Historical data over time further adds to the robustness of the door performance assessment.
Finally, data science and domain expertise individually cannot leverage the Sensor Fusion concept. Data scientists understand data modeling and can produce algorithms, but typically do not understand the lift domain. Conversely, domain experts can read lifts like a proverbial open book; however, they typically lack the knowledge and skills needed to model data and produce algorithms. Data scientists and domain experts need to work together to achieve success with Sensor Fusion.
5. Conclusion
Doors are among the busiest parts of the lift due to the nature of their purpose. With doors being present at every landing, they bring a multiplier effect in terms of overall lift maintenance effort. This also causes door-related failures to typically be among the most common causes for overall lift downtime, as earlier studies have shown.[1]
To further improve maintenance of lift doors, lift technicians need to be enabled to make better informed decisions by taking into account the unique variables of every individual lift, respectively the doors at every floor. This cannot be achieved without the aid of technologies such as IoT and ML while leveraging the concept of Sensor Fusion.
The concept of Sensor Fusion encompasses the simultaneous processing and wholistic interpretation of sensory inputs generated by multiple sensors in parallel. As such, Sensor Fusion resolves the prior technology limitations inherent to using the lift controller’s diagnostic data, which are limited to error codes and status mode changes. Sensors can be applied universally irrespective of the make, model or age of individual lifts. By using data directly taken from the sensor edge, data quality and usability limitations are negated by the ability to use the universal laws of physics.
When combining data science with domain expertise, actionable insights can be derived from the data. These actionable insights aid the lift technician in making better informed decisions on the actual maintenance needs of lift doors on a per-floor basis. Considering the aforementioned multiplier effect, significant optimization in terms of time spent on site can potentially be realized without compromising on the quality of service provided.
References
[1] A. Torres Perez, S. Kaczmarczyk and R. Smith, “Automatic Fault Detection and Classification in Lift Door Systems Using Vibration Signal Features”, 10th European Workshop on Structural Health Monitoring, EWSHM 2020 – Lecture Notes in Civil Engineering, Vol. 128, Springer, p.765-775 (2021).
[2] S. Freeman, “Who Invented the Elevator”, How Stuff Works, last updated March 12, 2024, accessible through science.howstuffworks.com/innovation/inventions/who-invented-the-elevator.htm, last accessed July 18, 2024.
[3] L. Gray, “A History of Elevator Doors”, ELEVATOR WORLD, published December 1, 2016, accessible through elevatorworld.com/article/a-history-of-elevator-doors/, last accessed July 18, 2024.
[4] B. Gustin, “Mechanical elevator door restrictors: what fire fighters need to know”, Fire Engineering, published August 1, 2003, accessible through fireengineering.com/leadership/mechanical-elevator-door-restrictors-what-firefighters-need-to-know/, last accessed July 19, 2024.
[5] K. Worden, J.M. Dulieu-Barton, An Overview of Intelligent Fault Detection in Systems and Structures. SHM, 2004, Vol 3(1), p.85–98.
[6] A Code of Safety Standards for the Construction, Operation and Maintenance of Elevators, Dumbwaiters, and Escalators – 1921. This Code of safety standards is intended as a guide for the construction, maintenance and operation of elevators, dumbwaiters, escalators and their hoistways, except as stated in the following paragraph. Rule 702 Maintenance. ASME, 1921.
[7] S. T. Park, B.S. Yang, “An implementation of risk-based inspection for elevator maintenance,” Journal of Mechanical Science and Technology, Vol. 24, No. 12, p.2367-2376 (2010).
[8] J. X. Yang, et al., “Real-Time Intelligent Elevator Monitoring and Diagnosis: Case Studies and Solutions with Applications Using Artificial Intelligence.” Computers and Electrical Engineering, Vol. 100, article 107965 (2022)
[9] A. Bassi et al., “Enabling Things to Talk”, Springer, DOI 10.1007/978-3-642-40403-0, p.1 (2013)
Get more of Elevator World. Sign up for our free e-newsletter.