The Most Important Escalator Safety Device
Dec 5, 2024
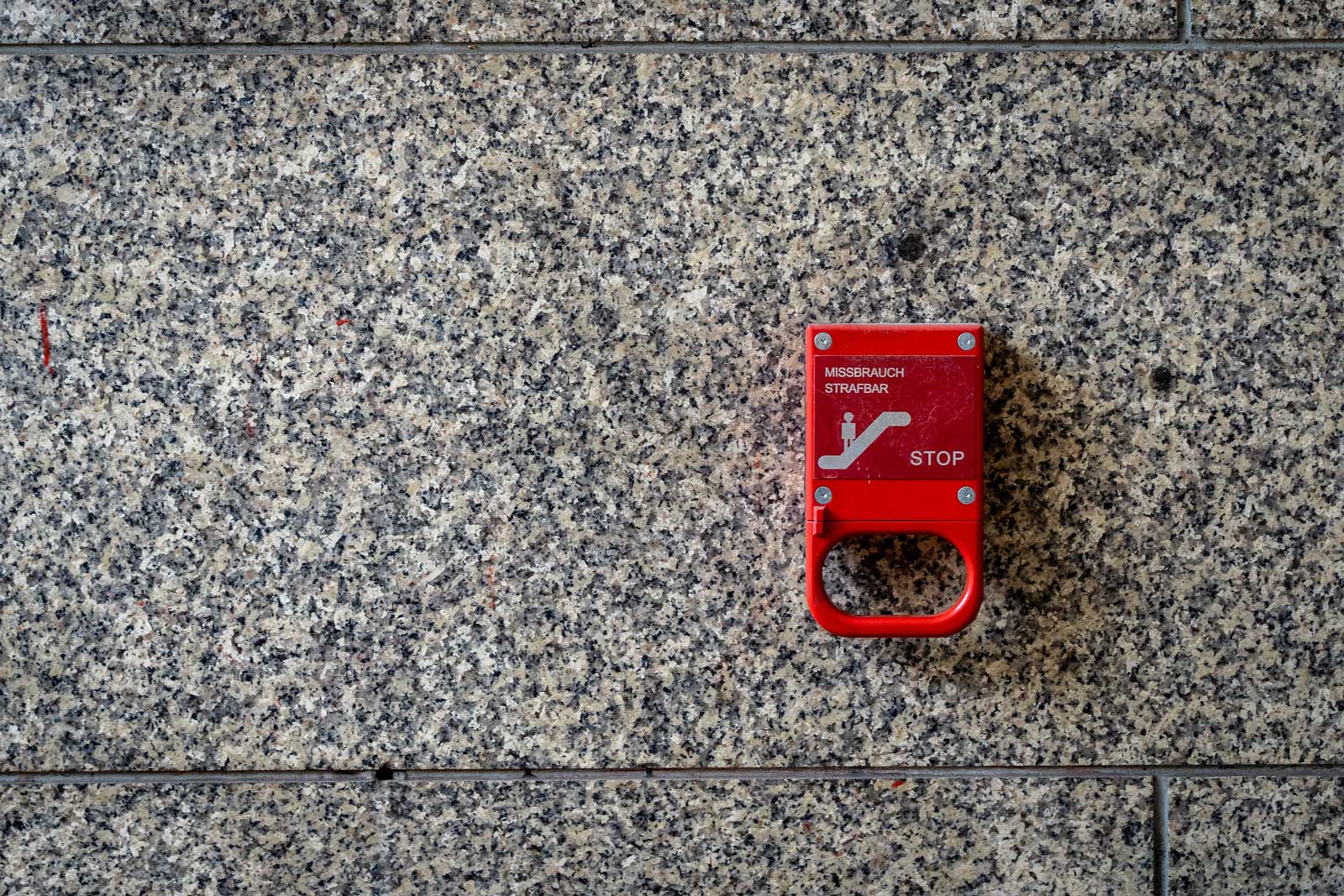
The evolution of escalator and moving walk braking systems
by Anthony Boom
Introduction
Ever since the very first escalator was installed, escalator manufacturers and safety code committees have worked proactively and reactively to make escalators as safe to ride as possible. Whether manufacturers and code committees were acting proactively or reactively, as time passed, many new safety devices and rules were implemented to make escalators and moving walks safer to ride.
In the more than 35 years that I have worked in the escalator business, I have seen the implementation of a variety of new safety devices such as missing step detectors, handrail speed sensors, comb impact devices, level step devices, overspeed and underspeed devices and more.
Much could be written about the addition and evolution of all escalator safety devices, but the focus of this article is on the evolution of the most important escalator safety device — the escalator brake.
The escalator brake is the utmost important escalator safety device since every other safety device on an escalator depends on the function of the escalator brake. If there were no escalator brake, then what would the value be of installing an emergency-stop switch or an overspeed device or a comb impact device or whatever safety device? The answer is, “none.” If there were no escalator brake, then the escalator would not stop when a safety device activated. At best, the escalator motor would shut off and the escalator would continue to move until the weight of the passengers on the escalator was insufficient to overcome the friction of the handrails and the rolling step band.
Fixed Torque Brakes and Flywheels
Early escalator brakes were simple spring-applied brakes, now referred to as fixed torque brakes. Tension springs and compression type springs were utilized to apply the escalator brake when required. Tension springs lost favor since the failure of the tension spring would result in having no brake at all, whereas a compression spring can still provide some spring force if the spring breaks.
Some drawbacks of a fixed torque brake:
- The brake must regularly be re-adjusted as the brake wears.
- If the fixed torque of the brake is adjusted such that the brake torque is sufficient to stop a heavily loaded escalator, then when the escalator is lightly loaded, the escalator may stop undesirably quick.
- The stopping distance of the escalator can vary greatly depending on the load on the unit. If the load on the escalator is great, then the escalator stopping distance will be long. Whereas, if the load on the escalator is light, then the stopping distance will be short.
To minimize the differences in escalator stopping distances and stopping rates, escalator manufacturers added a large flywheel to the escalator motor. Adding a flywheel to the escalator motor reduced the impact of the loading on the escalator. Essentially, the brake is working more to stop the flywheel rather than stopping the load on the escalator. The bigger the flywheel, the more consistent the stopping of an escalator becomes from no load on the escalator to a heavy load on the escalator.
Adding a flywheel not only makes the escalator stop more consistently, but the flywheel makes the escalator stopping rate more consistent in both directions — up and down. Without a flywheel mounted on the escalator motor, a load on the escalator will cause the escalator to stop short if the escalator is running in the up direction and stop long if the escalator is running in the down direction.
It may seem that the flywheel mounted on the escalator motor shaft rectified most of the issues of the fixed torque brake. However, adding a flywheel creates some new undesirable issues:
- The need for a much larger brake. With a flywheel mounted on the motor shaft, the brake must have enough stopping torque to stop the flywheel and the full rated load on the escalator.
- Increasing the size of the brake increases the cost of the brake.
- More space is required to mount the flywheel and the larger brake.
- A large electrical current is required to start the escalator motor and flywheel rotating and then accelerating to speed.
Escalator manufacturers have put up with these issues for decades to gain the benefits that adding a flywheel provides. However, as time went on, safety code requirements became more stringent. The code required a minimum stopping distance of 4.5 in. (4.5 in. was calculated on a maximum deceleration rate of 3 ft/s^2 for an escalator running at 90 ft/min.) Stopping quicker than 3 ft/s^2 was deemed to be destabilizing to passengers. However, the escalator had to be stopped in a distance no greater than about 16 in. This 16 in. was determined by the distance from the skirt switch to the comb teeth on the escalator. If someone’s shoe was entrapped between the edge of the step and the escalator skirt when tripping the skirt switch, the escalator had to be stopped before that person’s foot was dragged into the comb teeth.
To meet the code-allowed stopping distance range of 4.5 in. to 16 in., no matter the live load on the escalator, manufacturers experimented with increasing the size of the motor flywheel to minimize the effect of the live load on the escalator. Other manufacturers explored setting the brake torque high then delaying the application of the brake to meet the minimum stop distance requirement of 4.5 in.
Delaying the application of the brake helped meet the minimum stopping distance requirement, but failed to meet the maximum deceleration rate of 3 ft/s^2. So, the code committee added the requirement of setting the brake without delay to avoid a deceleration rate greater than 3 ft/s^2.
Variable Fixed Torque Brakes
In the 1980s, the company I worked for paired a permanent magnetic (PM) brake with a novel method of applying a fixed brake torque proportional to the load on the escalator. The advantage of the PM brake was and is that it is a variable torque device. The PM brake produces a torque that is inversely proportional to the current flowing though the PM brake coil. If the load on the escalator was heavy, then the brake controller could increase the brake torque by reducing the PM brake current. If the load on the escalator was light, then the brake controller could decrease the brake torque by increasing the PM brake coil current.
Now this brake controller determined the load on the escalator by measuring the phase angle between the escalator motor voltage and the escalator motor current. A larger phase angle implies a lighter load on the escalator, while a smaller phase angle implies a heavier load on the escalator. If the phase angle were small, then the escalator brake controller would reduce the PM brake coil current to apply more braking force while the escalator was stopping. When the phase angle was larger, the escalator brake controller would increase the PM brake coil current to decrease the braking torque.
Applying brake torque proportional to the load on the escalator eliminated the need for a flywheel while being able to meet the code requirements for deceleration rate and stopping distances. However, this brake controller required a fairly complex control setup that varied depending on motor size and motor manufacture. Additionally, the PM brake torque was not consistent from brake to brake for a given brake coil current. Consequently, additional setup adjustments had to be made from time to time or whenever the brake was replaced with a new brake.
Velocity Feedback Brake Control
In 1988, the same company came up with a new brake control that was simpler to set up and was independent from motor size, motor manufacture and variations in brake torque for a given brake coil current. This new method utilized “velocity feedback” with the same PM brake. The “velocity feedback” brake controller compared a predefined voltage ramp to the voltage of a tachometer driven by the escalator motor. The brake controller would vary the brake torque depending on how the tachometer voltage was following the predefined voltage ramp. If, while the escalator was stopping, the tachometer voltage was less than the predefined voltage ramp voltage, then the escalator was stopping quicker than desired. So, the brake controller would reduce the braking torque to decrease the stopping rate of the escalator. However, if the tachometer voltage was greater than the predefined voltage ramp, then the escalator was stopping slower than desired. So, the brake controller would increase the braking torque to decrease the stopping rate of the escalator.
The advantages of velocity feedback brake control included:
- Simplified set-up procedure
- Independent of motor size
- Independent of motor manufacture
- Independent of variations in brake torque for a given brake coil current
- Eliminated the need for a flywheel
As long as the brake could produce ample torque to stop the rated load, velocity feedback functioned fairly well.
The drawbacks of velocity feedback brake control included:
- The need to “tune” the tachometer to the predefined voltage ramp generated by the brake controller
- As the tachometer voltage degraded over time, the tachometer had to be “retuned” to the predefined voltage ramp generated by the brake controller.
- The brake controller had only one preprogrammed voltage ramp to which the tachometer feedback voltage was compared. So, different brake control cards were required for each speed that an escalator or auto walk was designed to run.
It should be noted that, to prevent an unsafe situation, the escalator controller would not allow the escalator to run if the voltage on the tachometer was too low.
Deceleration Feedback Brake Control
In 1993, the same company came up with another new brake controller that had all the advantages of the “velocity feedback” brake controller but was even simpler to set up and did not have to be “tuned” or “re-tuned.” This new method utilized “deceleration feedback” with the same PM brake. This brake controller utilized an encoder instead of a tachometer to determine velocity and to calculate real-time escalator deceleration. The calculated real-time escalator deceleration rate was then compared to a desired deceleration setpoint. The brake controller utilized a PI (Proportional and Integral) control to vary the brake torque depending on how close the real-time escalator deceleration rate was to the desired set point. If, while the escalator was stopping, the real time escalator deceleration rate was greater than the setpoint, then the escalator was stopping quicker than desired. So, the brake controller would reduce the braking torque to decrease the stopping rate of the escalator. However, if the real-time escalator deceleration rate was less than the setpoint, then the escalator was stopping slower than desired. So, the brake controller would increase the braking torque to increase the stopping rate of the escalator.
The advantages of the deceleration feedback brake control include:
- All the advantages of the velocity feedback controller
- An even simpler set-up procedure
- No tuning or retuning
- The same brake control card can be utilized on any speed escalator or auto-walk.
Other improvements built into the new deceleration feedback brake controller included:
- The use of PWM (Pulse Width Modulation) instead of linear regulation to control brake current. PWM provides more precise control and is more energy efficient than the older linear control.
- The use of PI control to regulate the brake coil release current was added. So, as the brake coil resistance varied from brake to brake, or as the brake coil resistance varied due to temperature variations, the PI controller would maintain the same brake release current over time.
- The fuzzy logic that I received a patent on for brake control estimated the load on the escalator so that the initial brake torque closely matched actual required stopping torque. The result of estimating the load on the escalator before the brake engaged reduced hunting time and magnitude to produce a smoother brake engagement.
As long as the brake could produce ample torque to stop the rated load, the deceleration feedback functioned well.
One of the major drawbacks of deceleration feedback brake control is on an up-running escalator. A loaded up-running escalator will always stop quicker than the desired deceleration setpoint if there is no flywheel mass mounted on the escalator motor. However, at this time, the Escalator Safety Code does not require a maximum deceleration rate when the escalator is running in the up direction.
Motor-Controlled Dynamic Braking
Earlier in 2024, I finished testing a new hybrid braking system for escalators and moving walks. This hybrid braking system incorporates deceleration feedback braking control with another type of brake control recently permitted by the ASME A17.1-2019 and 2022 Escalator Safety Code Rule 6.1.5.3.4. The Escalator Safety Code refers to this new braking system as “Motor-Controller Dynamic Braking.” Motor-controlled dynamic braking requires power to remain connected to the escalator motor during the braking process because the motor is being utilized for braking.
Actually, motor-controlled dynamic braking is not new, but until recently, was not permitted for use on escalators and moving walks because the Escalator Safety Code has always required the removal of power from the driving machine motor and brake whenever a safety device is activated. The Escalator Safety Code now permits the use of motor-controlled dynamic braking, provided several other rules are followed.
The advantages of motor-controlled dynamic braking:
- All the advantages of the deceleration feedback controller
- The escalator does not speed up or slow down while waiting for the brake to engage when a safety device is actuated. Without dynamic braking, the escalator will “freewheel” during the time it takes from a normal brake to move from open to engaged. While normal brake engagement time is relatively small, the escalator will speed up momentarily under load in the down direction until the brake engages. With dynamic braking, the freewheeling of the motor is eliminated. Consequently, the stopping process is improved.
- Consistent stopping rate regardless of load on escalator or running direction
- Consistent stop distances regardless of load on escalator or running direction
- Can be used as an additional means of stopping the escalator — Code required above 6 m
- Eliminates need for second machine brake when a redundant brake is required by code
- The stopping profile is smooth as a fixed torque brake.
The drawbacks of motor-controlled dynamic braking included:
- Motor cannot produce torque for braking on power loss.
- The Escalator Safety Code permits the use of motor-controlled dynamic braking for most safety devices but not all. Dynamic braking is NOT permitted for escalator speed monitoring, drive chain device, pit switches, stop reversal, disconnected motor safety device, comb-step impact device and several more.
To overcome the disadvantages of motor-controlled dynamic braking, I paired dynamic braking with deceleration feedback control to form a hybrid system. For most safety devices, motor controller dynamic braking is utilized. Where motor-controlled dynamic braking cannot be utilized, the hybrid system utilizes deceleration feedback control.
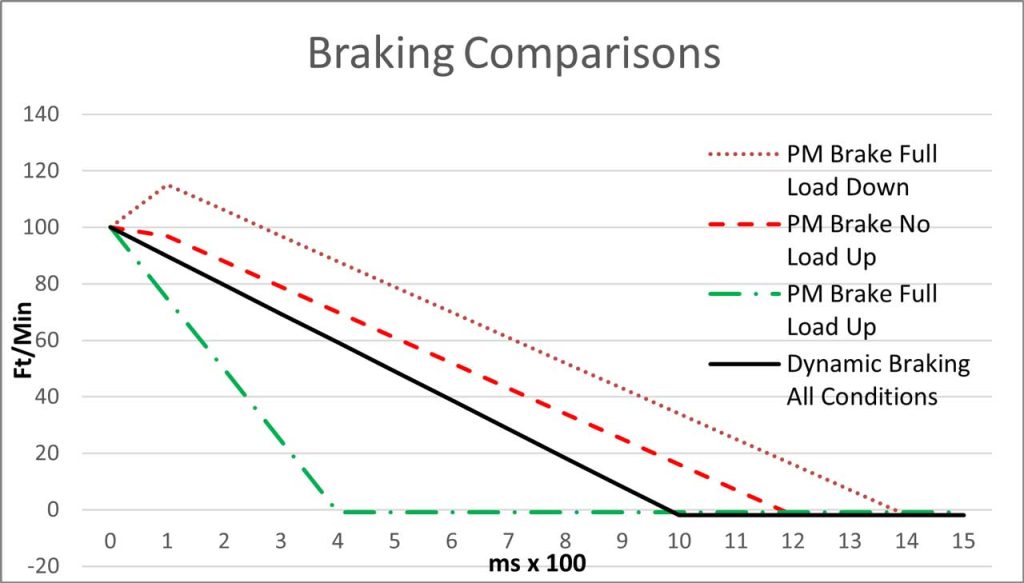
Braking Comparison Chart
- Top dotted line represents PM braking full load down direction.
- Second-from-the-top dashed line represents PM braking no load on escalator either direction.
- Middle solid line represents dynamic (regenerative) braking, all conditions, loaded, unloaded, up direction, down direction.
- Bottom dashed line represents PM braking full load up direction.
- With any spring or magnetically applied brake there is an application time. The braking comparison chart shows a brake application time of 100 m/s and an escalator run speed of 100 ft/min. The actual speed of the escalator depends on the actual no load run escalator speed, running direction and the load on the escalator. A loaded up-running escalator will run slower than the actual no-load escalator speed and a loaded down-running escalator will run faster than the actual no-load escalator speed.
- The full load PM braking down direction graph shows that the speed of the escalator increased at 2.5 ft/s2 from 100 ft/min to 115 ft/min and moved 2.15 in. before the brake engaged. Then, the PM Brake controller controlled the deceleration rate to 1.5 ft/s2 for an additional 14.7 in. Total stopping time is about 1.4 s with a total stop distance of 16.8 in. If there were zero application time and if there were sufficient braking torque to stop the load at 1.5ft/s2, then the actual stop distance would be 11.11 in.
- The no-load PM braking graph shows that the speed of the escalator decreased at 0.5 ft/s2 from 100 ft/min to 97 ft/min and moved 1.97 in. before the brake engaged. Then, the PM brake controller controlled the deceleration rate to 1.5 ft/s2 for an additional 10.45 in. Total stopping time is about 1.2 s with a total stop distance of 12.4 in. Depending on the inertia and friction of the mechanical system, the actual time, stop time and distance will vary. If there were zero application time and if there were sufficient inertia to overcome the system friction, the actual stop distance would be 11.11 in.
- The full-load PM braking up-direction graph shows that the speed of the escalator decreased at 4.2 ft/s2 from 100 ft/min to 74.8 ft/min and moved 1.75 in. before the brake engaged and then continued to decelerate at 4.2 ft/s2 for an additional 2.22 in. Total stopping time is about 0.4 s with a total stop distance of 3.97 in. In this case, the PM Brake controller will feather or apply little braking force. The weight on the escalator will slow the escalator to a stop quicker than the programmed rate of the brake controller. Total stopping time is about 0.4 s with a stop distance of 3.9 in. Depending on how much weight is on the escalator and the inertia and friction of the mechanical system, the actual time, stop time and distance will vary.
- The dynamic (regenerative) braking graph shows no delay time in braking. Regardless of the load on the escalator and running direction, the graph shows the escalator decelerated at 1.7 ft/s2 from 100 ft/min to 0 ft/min in 1.0 s in a total stop distance of 9.8 in. In this case, the PM brake controller will feather or apply little braking force during stopping since the inverter is slowing the escalator faster than the set point of the brake controller. In the up direction, the inverter will “push” the escalator load to maintain the 1.7 ft/s2 deceleration rate.
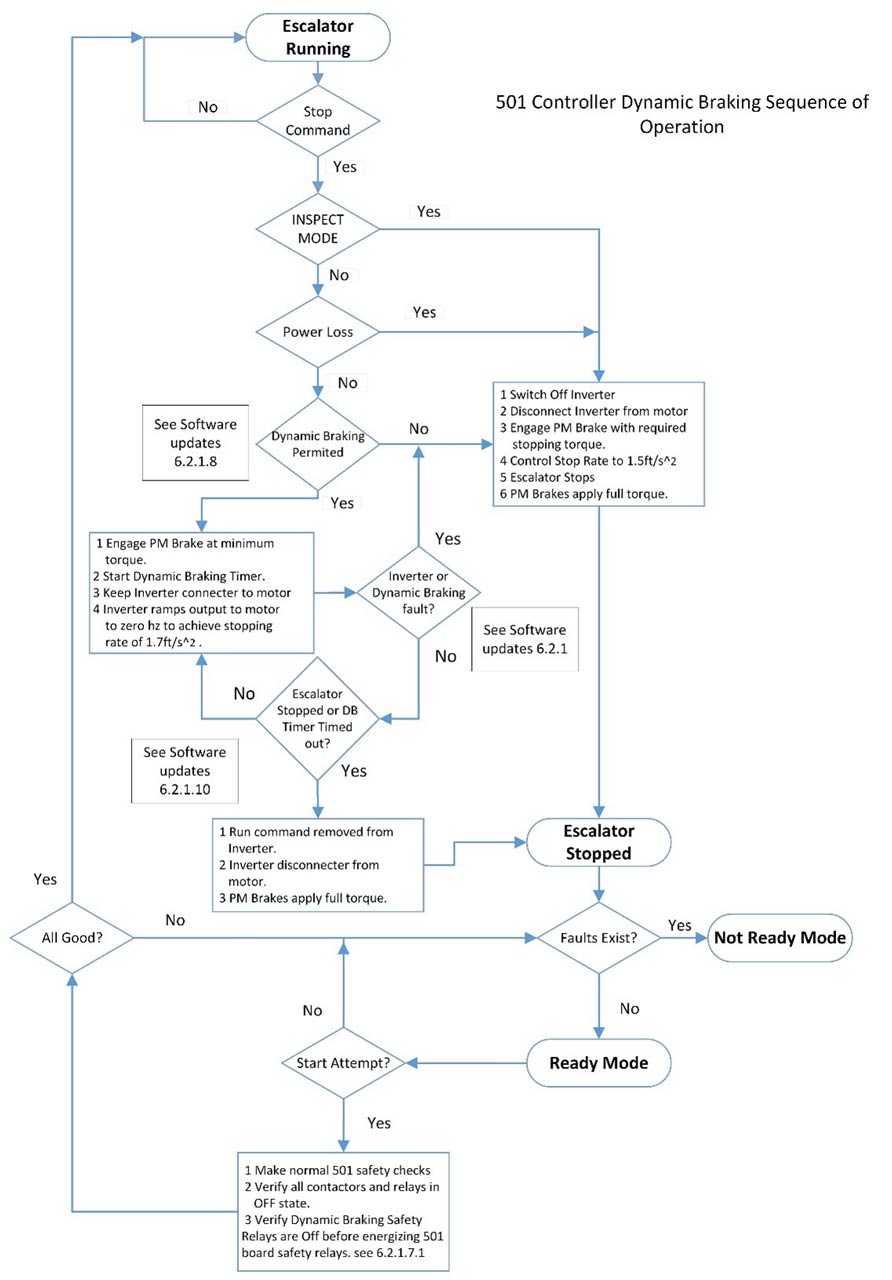
Conclusion
Ever since the very first escalator was installed, escalator manufacturers and safety code committees have worked proactively and reactively to make escalators and moving walks as safe as possible by adding and improving safety devices.
The most important safety device on the escalator is the escalator brake. All other safety devices depend on the escalator brake to stop the escalator when a safety device is actuated. The brake must not only stop the escalator or moving walk, but the brake must stop the escalator in a manner that is as safe as possible.
As previously discussed, escalator brakes started out as fixed torque brakes. Then improvements came with the addition of flywheels, variable fixed torque brakes, velocity feedback, deceleration feedback and, now, motor-controlled dynamic braking.
The braking system I recently finished testing is a hybrid system that combines motor-controlled dynamic braking with deceleration feedback braking for when motor-controlled dynamic braking cannot be utilized.
Code References
ASME A17.1-2019/CSA B44:19 Rule 6.1.5.3 Brakes
- 6.1.5.3.1 Escalator Driving-Machine Brake
- 6.1.5.3.2 Main Driveshaft Braking
- 6.1.5.3.3 Escalator driving-machine brakes and motor-controlled dynamic braking systems shall be certified to the requirements of 8.3.1 and 8.3.6
- 6.1.5.3.4 Escalator Driving-Machine Motor-Controlled Dynamic Braking
- 6.1.5.3.5 Auxiliary Brake
- ASME A17.1-2019/CSA B44:19 Rule 6.1.6.7 Escalator Braking-Distance Monitor
- ASME A17.1-2019/CSA B44:19 Rule 6.1.6.3 Electrical Protective Devices 6.1.6.3.1 through 6.1.6.3.16 include the following verbiage if dynamic braking is permitted for the device, “shall cause initiation of dynamic braking (6.1.5.3.4) or the electric power to be removed from the escalator driving machine motor and brake.” If dynamic braking is not permitted, then the following verbiage will be omitted: “shall cause initiation of dynamic braking.” The devices are enumerated in the Requirements section of this document.
- ASME A17.1-2019/CSA B44:19 Rule 6.1.6.10 Control and Operating Circuits. The design and installation of the control and operating circuits shall conform to 6.1.6.10.1 through 6.1.6.10.4.
- ASME A17.1-2019/CSA B44:19 Rule 8.3.1 General Requirements for Tests and Certification
- ASME A17.1-2019/CSA B44:19 Rule 8.3.6 Escalator Brake and Braking System Type Tests
Get more of Elevator World. Sign up for our free e-newsletter.