Using Circular Economy Business Models and Life Cycle Assessment to Improve the Sustainability of Elevators
Apr 6, 2023
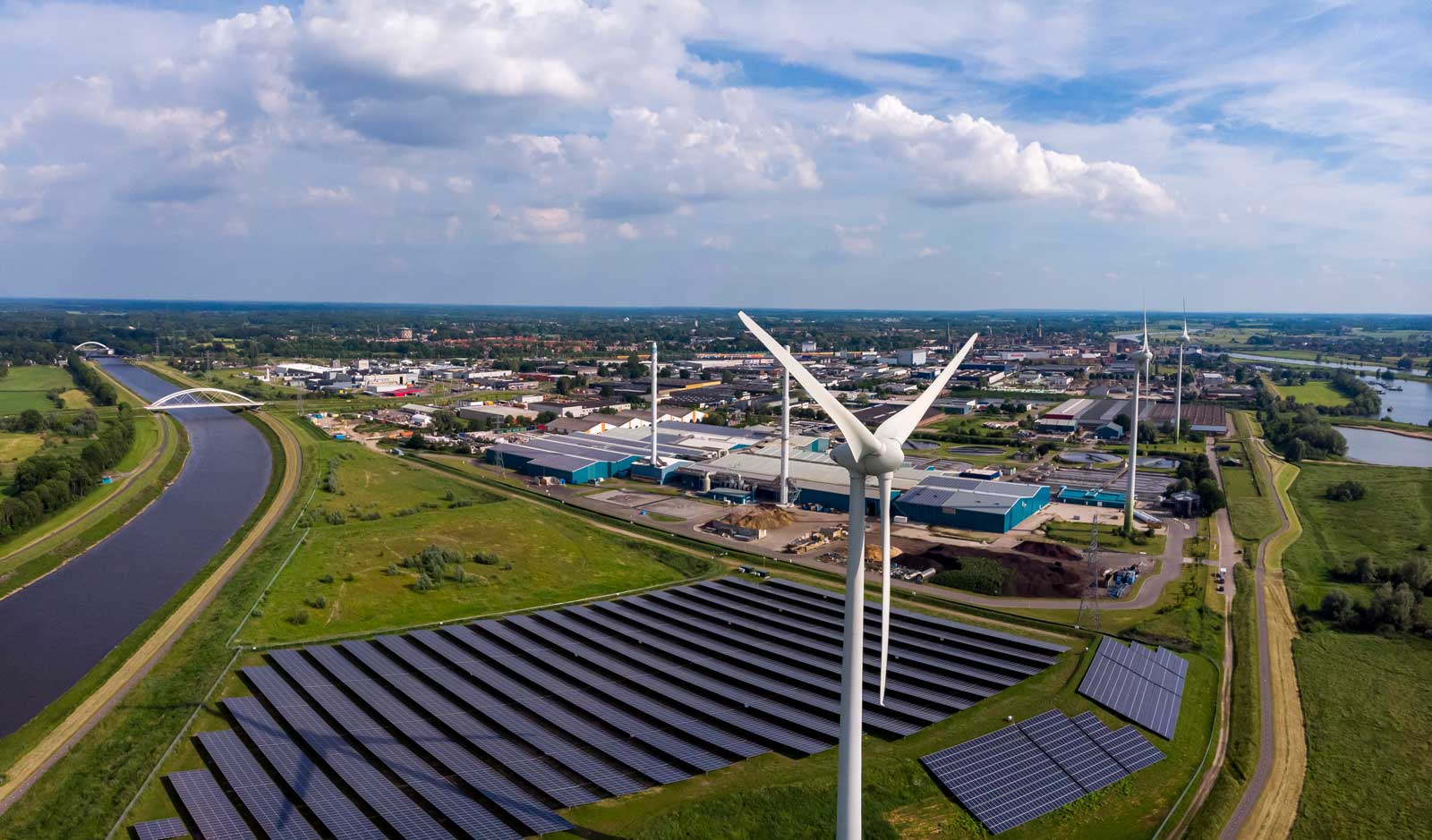
Research explores the potential of drive refurbishment to improve elevator sustainability.
by Marco Tomatis, Christian Kukura, Siniša Djurović, Judith Apsley, David Griffin, Jordan Griffin, Rob Corner and Laurence Stamford
Abstract
About 55% of the world population is currently living in cities, and an increasing level of urbanization is expected in the future. Therefore, construction of tall buildings is increasing and, accordingly, the installation and maintenance of elevators are increasingly crucial enablers. Electric drives are one of the main components of elevators, controlling their movement, speed and torque. The lifetime of elevator drives is commonly shorter than that of the elevator, and thus, the drive needs replacing one or more times during the elevator’s lifetime, contributing to environmental impacts. This work explores the potential of drive refurbishment to improve the sustainability of the elevator. The potential reduction of environmental impacts related to the use of refurbished compared to new drives was assessed via life cycle assessment (LCA). Two elevator drives, the OVF20 (Otis) and the VF22BR (Schindler), were considered for this assessment, including empirical data collection on component materials and masses. Across a range of 18 impact categories, the results showed that refurbishing an elevator drive results in 53-91% lower environmental impacts compared to the construction of a new drive and has the potential to reduce e-waste volumes by 72-84% depending on the drive considered. Across the lifetime of a whole elevator system, electricity consumption is the main source of impacts, accounting for 63-99% of the total, while the drive itself contributes ≤27%. Nonetheless, it is possible to decrease the lifetime environmental impacts of an elevator by 1-17% by installing refurbished elevator drives. Overall, the results of this study suggest that the use of circular economy strategies can markedly improve the environmental sustainability of elevators and could be coupled with other green elevator strategies to enable more eco-friendly urbanization.
Keywords: Lifts; Circularity; LCA; E-waste; Environmental impacts; Green elevator strategy; Elevator drive
1. Introduction
Significant increases in urban population have been reported during the past 30 years, with about 55% of the global population currently residing in cities,[1] and forecasts reporting that this trend is expected to continue.[2] This rapid urbanization has led to the construction of increasingly taller buildings to optimize land use in urban areas.[3] In this context, elevators are crucial to maintain the accessibility of tall buildings. Consequently, since 2012, the number of elevators installed worldwide has increased from about 11 million to more than 18 million,[4] with continued growth expected in the foreseeable future: the market is predicted to grow from its current value (US$99.30 million) to US$120-130 million by 2029.[5, 6]
A variety of prior scientific literature on elevators has addressed performance, energy efficiency, maintenance and control and other predominantly technical issues.[3, 7-10] Elevator drives are one of the main components of elevators as they control their movements, speed, position and torque. When these drives malfunction, they are typically replaced with new ones, and the defective drives are disposed as e-waste. Moreover, the lifespan of an elevator drive, which varies depending on the drive model, is commonly shorter than that of the elevator itself (the latter being at least 20-30 years).[8, 11] Thus, it is possible that the drive will be substituted multiple times during the lifespan of the elevator. Consequently, refurbishing malfunctioning elevator drives could greatly reduce the amount of e-waste produced. However, to the authors’ knowledge, there are no prior publications investigating the environmental sustainability implications of such a circular strategy in the elevator sector.
Accordingly, this study uses LCA to investigate the impact and footprint of elevator drive refurbishment according to the procedures developed by a U.K.-based company — Northern Drives and Controls (NDC) Ltd. — and compares this to the standard industry practice of installing new replacement drives. Two different commercial elevator drives, namely the OVF20 (Otis) and the VF22BR (Schindler), are used as exemplars. In order to provide a broader context to the impacts of an elevator drive, its environmental sustainability is also assessed as a part of the whole elevator system. The aim of this assessment is to determine the potential benefits of applying waste management options which adhere to circular economy principles for elevator drives. More broadly, this study aims to provide further foundations for circular business models in sectors dealing with electronics and/or urban infrastructure.
2. Methodology
The LCA models were developed according to the ISO 14040/44 guidelines,[12, 13] and thus, the goal and scope of the study, inventory analysis, impact assessment and interpretation are described below. All assessments followed the attributional approach, and GaBi 10.5 software[14] was used for system modeling.
2.1 Goal and Scope
The main goal of this study was to assess the environmental impacts of refurbishing elevator drives in comparison to the installation of new ones. To account for variability between drives, two different models were chosen to be analyzed side by side: Otis OVF20 and Schindler VF22BR. These specific models were chosen based on their prevalence in the market, as determined by the direct experience of project partner NDC Ltd.
The purpose of this study was to allow the identification of benefits and drawbacks of refurbished drives, as well as improvement opportunities. A secondary goal was to assess the environmental sustainability of the drive in the context of the whole elevator system to determine the relevance of the elevator drive to the impacts of the elevator.
The functional unit (FU) for the elevator drive is “refurbishing or production of a drive unit,” while for the LCA of the elevator system, the functional unit is “25 years of operation of the elevator,” which represents the average lifetime of an elevator.[8, 11, 15]
A cradle-to-grave approach was considered for the production/refurbishing of the elevator drive (Figure 1). The system boundaries for the refurbishing process include the drive’s transportation to the repair site, testing to assess which components need to be replaced, repairing, a second set of tests to assess if the refurbished drive is performing as expected, disposal of e-wastes produced and transport of the refurbished drive to the elevator site. A simpler system was considered for the assembly of a new drive, which included the assembly of a new drive, its transportation to the elevator site and the disposal of the broken drive. For the LCA of the elevator system, the lifetime electricity consumption of the elevator was also accounted for.
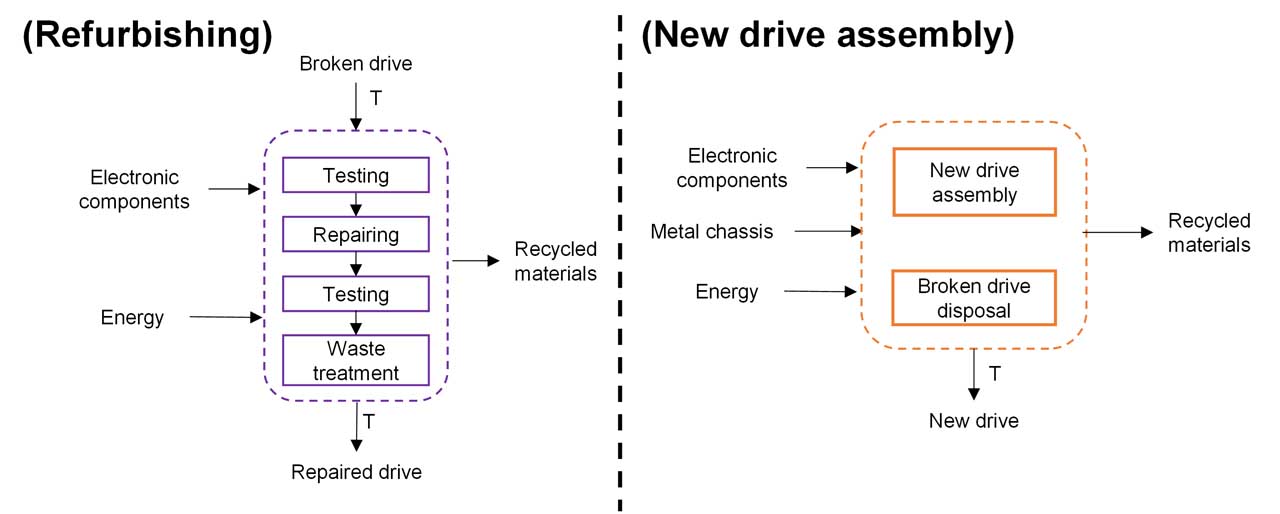
2.2 Inventory Data
Tables 1 and 2 report inventory data for the OVF20 and VF22BR drive, respectively. Inventory data for the refurbishing processes were provided directly by project partners NDC Ltd., while the balance of materials for the drives themselves was estimated empirically by disassembling each drive and weighing and identifying its components. This was performed at The University of Manchester Power Conversion Laboratory, where each assessed drive was methodically taken apart following commercial guidelines and the required characteristics of its subassemblies evaluated. Background data for materials and energy were obtained from Ecoinvent 3.7,[16] assuming that the refurbishing process is based in the U.K., while the production of new drives depends on the location of the producer company. The authors note that some of the inventory data on electronics in the Ecoinvent database originally dates from the 2000s and may require updating to accurately reflect current electronics manufacture. However, in the absence of newer, robust data, it remains the most reliable source.
For the transportation of new and refurbished drives, it was assumed that 60% of the broken drives would come from the U.K., 30% from Europe and 10% from the U.S., based on real market data from NDC Ltd. For the refurbished drive, it was assumed that drives from the U.K. could be transported to the refurbishment site by road, while flights would be necessary for other locations based on the quick turnaround time expected by elevator owners. Return trips to the elevator site were also accounted for. Bulk transportation by shipping to warehouses located in different countries was considered for new drives, with short haul flights or road transportation assumed to transport the drives from the warehouse to the elevator site. A transportation distance of 50 km to the disposal site was considered for all waste treatment. The percentages of different materials recycled or landfilled are estimated according to country-specific statistical data and relevant regulations.[17-21]
For the estimation of the elevator’s electricity consumption an energy calculator provided by TK Elevator was used.[22] For this case study, it was assumed that the drive unit was part of a geared office elevator with capacity for six people, serving a six-floor building and operating at a speed of 0.8 m/s. Accordingly, an electricity consumption of 89.9 MWh over 25 years of operation was estimated. The U.K. electricity mix was adopted for this case study, using the latest version (circa 2017) available in Ecoinvent: 39% natural gas, 22% nuclear, 19% renewables (wind, solar, hydro), 8% imports, 6% biomass and 6% coal. Given the continued greening of the electricity supply, the use of a recent mix is conservative. Note that electricity consumption from the exhaust fan and from the cab lighting have been excluded from this total as these are independent from the elevator drive and are unlikely to contribute significantly to the total. The rated power consumption of the two drives under investigation during operation is 9 and 2 kW for the OVF20 and VF22BR drive, respectively.
Itema | OVF20 | |||
| New | Refurbished | Unit per FU | Comment |
Chassis | 14.1 | – | kg | Mainly aluminium |
Printed circuit board (PCB)b | 0.15 | – | m2 | Includes four PCBs |
Transformer | 409 | – | g | Includes one transformer mounted on a PCB |
Resistor | 201 | – | g | Includes 19 resistors mounted on PCBs |
Capacitor | 1.1 | 1.0 | kg | Includes electrolytic and film capacitors mounted on PCBs. An average of 33 out of 34 capacitors are replaced during refurbishment |
Power line filterc | 2.8 | – | kg | Includes 1.1 kg of chassis and 1.7 kg of electronic components |
IGBT module | 1.5 | 1.0 | g | Includes four IGBT modules. Three of the four modules are substituted during refurbishment |
Optocoupler | 5.6 | – | g | Includes seven optocouplers mounted on PCBs |
Cooling fanc | 1.9 | – | kg | A single metal fan is used for cooling |
Contactor | 1.6 | – | kg |
|
Cable block | 745 | – | g |
|
Current transducerc | 36 | 36 | g | Includes two transducers mounted on PCBs |
Transient surge absorber | 3.9 | – | g | Includes two surge absorbers mounted on PCBs |
Gas discharge tube | 2.7 | – | g |
|
Fuse | 4.6 | 4.6 | g |
|
Testing electricity | – | 0.44 | kWh |
|
Elevator lifetime electricity consumption | 89.9 | 89.9 | MWh |
|
Transport | 155 | 65.4 | tkm | Transportation distances based on locations of Otis and NDC headquarters |
b The PCB production process is based on literature data [23].
c Background process from Ecoinvent was modified to describe the specific component.
Table 1: Inventory data for the production and refurbishing of the OVF20 drive [FU = functional unit]
2.3 Impact Assessment
The environmental impacts were estimated using the ReCiPe 2016 V1.1 impact assessment method at the mid-point level, following the hierarchist approach.[24] Seven impact categories, including climate change, depletion- and toxicity-based categories, were selected for this assessment from the 18 impact categories of the ReCiPe method due to their relevance to the assessment and their representativeness of the trends observed.
3. Results and Discussion
Figure 2 reports the life cycle impacts estimated for the OVF20 and VF22BR drives. Note that, for each drive, the refurbished and new cases are compared on a 1:1 ratio. This is conservative because the refurbishment process addresses common points of failure for each drive model, meaning that a refurbished drive may, in fact, last longer than a new one, leading to a smaller number of refurbished drives being required over the lifespan of the elevator compared to new drives.
Figure 2 (a) reports the life cycle impacts of the OVF20 drive, demonstrating that refurbishment causes lower environmental impacts (by 53-83%) than the production of a new drive. In both cases, impacts are mainly caused by the materials and energy used in the drive’s production or refurbishing (66-90% and 55-95% of the total impact, respectively).
In the case of the refurbished drive, transport shows significant contributions to climate change (23%), fossil depletion (22%) and terrestrial ecotoxicity (15%), while it emerged as a minor contributor to human toxicity (2%), and a negligible contributor (<1%) to other impacts. On the contrary, transport emerged as a minor contributor to the environmental impacts of the new drive, accounting for ≤2% in all categories considered. The larger contribution of transport to the impacts of the refurbished drive is because transportation to the refurbishment site and a return trip to the elevator site was included, with longer hauls being performed by flight; in contrast, one-way transport and different assumptions were assumed for the new drive (Section 2.2).
Recycled materials were credited to the system as part of the waste treatment, subsequently decreasing the environmental impacts of the refurbished drive by 2-30%. The recycling credits applied to the new drive were more substantial, lowering the impacts by 10-32%, mainly because of the recycling of the aluminum chassis (which would not be disposed of during refurbishment). Although more significant credits are attributed to the waste treatment of the new drive, it should be borne in mind that more wastes are produced, including 17.1 kg of metals and 7.6 kg of e-waste, in comparison to the refurbished drive (2.1 kg of e-waste), which could result in larger costs for waste treatment.
Similar trends were found for the VF22BR drive, with refurbishment resulting in 61-91% lower environmental impacts than those of a new drive (Figure 2 b). Drive refurbishing (57-91%) or production (57-89%) emerged as the main contributors to the impacts in both cases, while transport shows significant contribution to the impacts of the refurbished drive on climate change (25%), fossil depletion (23%) and terrestrial ecotoxicity (14%), but negligible contributions to other categories. For the new drive, transport accounts for ≤5% of the impacts. Credits from the recovery of recycled materials allowed a reduction in impacts of both refurbished (2-29%) and new drives (10-38%). The greater credits observed for the new drive are due to the larger mass of waste produced by its disposal (12.1 kg of metal components and 13.4 kg of e-wastes per drive) compared to the disposal of refurbished components (2.1 kg of e-wastes per drive). Accordingly, the refurbishing of VF22BR drives enables a 92% reduction in the total amount of waste produced and an 84% reduction in the amount of e-waste.
Overall, this analysis clearly shows the potential benefits of drive refurbishment in comparison to replacement with a new drive. However, the environmental impacts of the refurbished drive could be reduced further by optimizing the logistics to reduce transportation distances and avoid, where possible, air freight. To this end, the authors note that NDC is currently setting up service centers in various key countries, such as the U.S., Germany and Spain, which should enable logistics streamlining and therefore impact reduction. Ultimately,
a robust comparison of real-world transportation differences between new and refurbished drives is difficult to establish due to differing business practices, market exposures and other variables across the sector.
Itema | VF22BR | |||
New | Refurbished | Unit per FU | Comment | |
Chassis | 11.6 | – | kg | Mainly aluminium |
PCBb | 462 | – | cm2 | Includes two PCBs |
Transformer | 4.1 | – | kg | Includes two transformers in the chassis and two smaller transformers mounted on PCBs |
Resistor | 888 | – | g | Includes a resistor in the chassis and six smaller resistors mounted on PCBs |
Capacitor | 871 | 871 | g | Includes electrolytic and metal film capacitors mounted on PCBs |
Power line filter c | 1.1 | – | kg |
|
IGBT module | 277 | 277 | g | Includes two IGBT modules |
Power supply | 240 | 240 | g |
|
Cooling fan | 345 | 345 | g | Three plastic fans are used for cooling |
Contactor | 337 | 337 | g |
|
Cable block | 17 | – | g |
|
Safety relay | 35 | 35 | g |
|
Testing electricity | – | 0.278 | kWh |
|
Elevator lifetime electricity consumption | 89.9 | 89.9 | MWh |
|
Transport | 62.4 | 67.6 | tkm | Transportation distances based on locations of Otis and NDC headquarters |
b The PCB production process is based on literature data [23].
c Background process from Ecoinvent was modified to describe the specific component.
Table 2: Inventory data for the production and refurbishing of the VF22BR drive [FU = functional unit]
3.1 Assessment of the Elevator System
Figure 3 reports the environmental impacts of the whole elevator system and shows that the lifetime electricity consumption of the elevator is the main source of impacts for refurbished (89-99%) and new drives (63-99%), for both drive models. Larger contributions are estimated for the new drive (1-27%) compared to a refurbished one, but in both cases the drive itself shows negligible contributions to climate change, fossil depletion and water depletion, as well as ≤8% to the other impacts. This is because, based on available data, there is no reason to expect any difference in the electricity consumption of elevators utilizing new versus refurbished drives, and therefore, electricity is similarly dominant in both cases. Overall, this analysis shows that utilizing refurbished drives has the potential to reduce the life cycle impacts of the elevator by 1-17% for the OVF20 and by 1-16% for the VF22BR.
It should be noted that, during the lifespan of an elevator system, the supplied electricity mix is likely to become lower carbon with a greater contribution from renewables. Consequently, the relative contribution of electricity to the environmental impacts is likely to decrease over time, meaning the relative importance of the drive and other hardware will increase, along with the benefits derivable from refurbishment.
4. Conclusions
This study assessed the potential cradle-to-grave environmental benefits of refurbishing elevator drives as a circular economy strategy, contrasting with the conventional practice of replacement with a new drive unit. In a one-to-one comparison between drives, the results show that refurbished drives have the potential to reduce the total amount of waste produced by 91-92% and the amount of e-waste by 72-84% depending on the drive model. It was also observed that the reduction in material usage achievable via the refurbishment strategy enables a decrease in the drive’s environmental impacts of 53-91%, compared to the production of a new drive. When considering the whole elevator system, it was found that electricity use is the main contributor to the impacts (63-99%). However, elevators using refurbished drives have the potential to reduce their life cycle impacts by ≤17% due to the reduced mass of materials needed. These findings are conservative as, depending on the drive model and refurbishment process, the refurbished drive might have a longer lifespan than a new drive due to the elimination of common failure points. Consequently, the above results indicate the minimum likely benefits of a repair-oriented circular business model.
Overall, refurbishment offers the potential to significantly reduce the demand for materials and the generation of waste during the lifetime of elevators, improving their environmental sustainability significantly. Future research should address the optimization of transport logistics for elevator drives, and the application of circular models to emerging drive technologies, as well as other components of elevator systems.
David Griffin, managing director, NDC, said:
“We have always believed that our model — of high-quality drive refurbishment — was not just a cost effective opportunity for clients but a sustainable approach that made the most of existing units and materials. Refurbishment is a true alternative to purchasing new drives.”
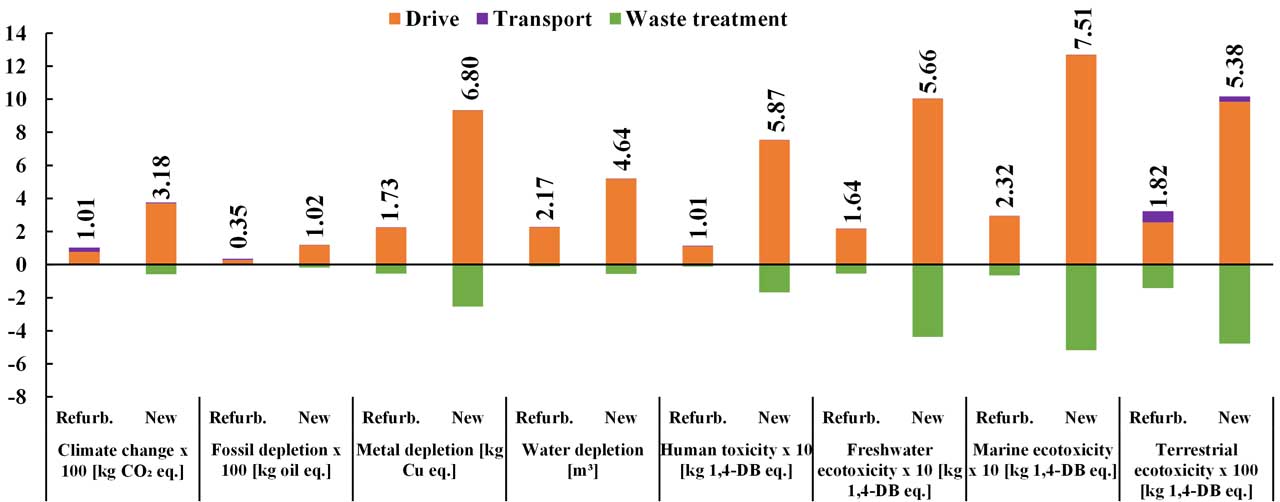
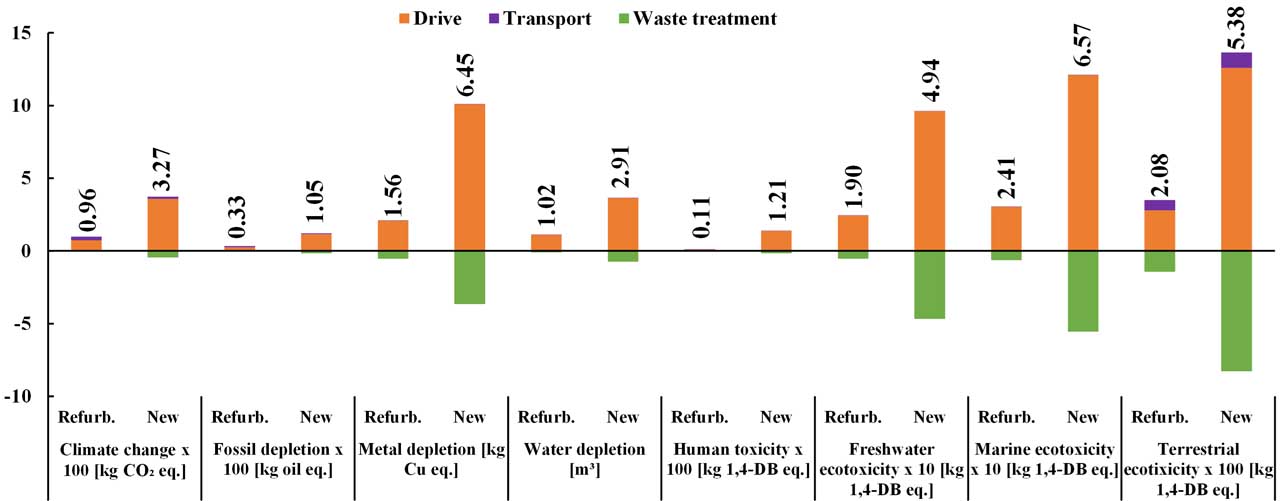
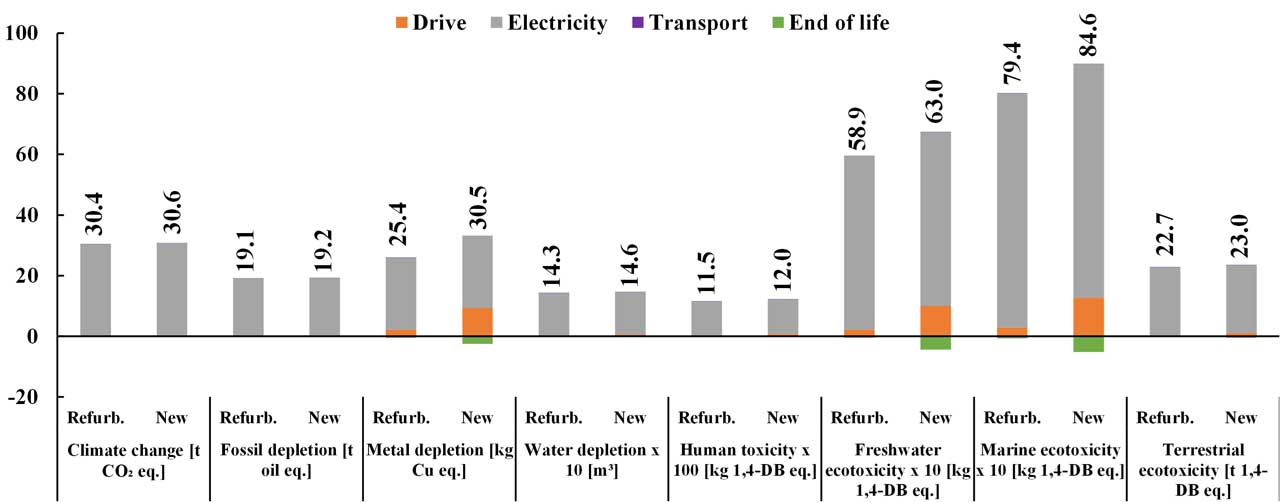
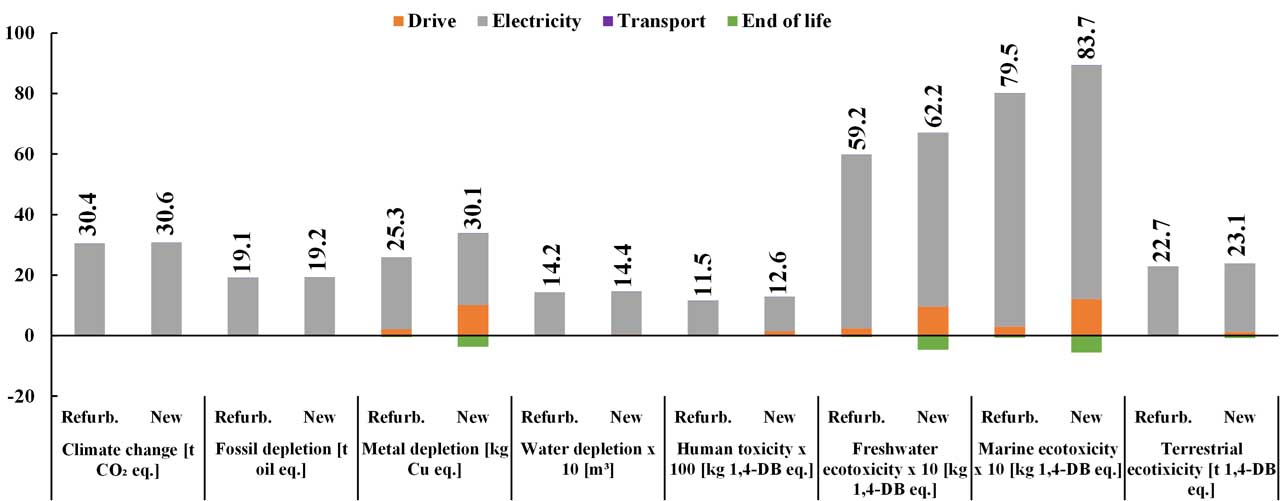
Acknowledgments
The authors gratefully acknowledge the funding provided by the UK Research and Innovation Impact Acceleration Account via The University of Manchester.
References
[1] Urban development overview; The World Bank, worldbank.org/en/topic/urbandevelopment/overview#1. Accessed: June 2022.
[2] K.C. Seto, B. Guneralp, L.R. Hutyra, Global forecasts of urban expansion to 2030 and direct impacts on biodiversity and carbon pools. Proc Natl Acad Sci U S A, 109, pp. 16083-16088, 2012.
[3] Z. Dalala, T. Alwahsh, O. Saadeh, Energy recovery control in elevators with automatic rescue application. Journal of Energy Storage, 43, 2021.
[4] Number of elevators and escalators in operation from 2012 to 2021; Statista, statista.com/statistics/1201896/elevators-escalators-operation-worldwide/. Accessed: June 2022.
[5] Global Elevator Market – Industry Trends and Forecast to 2029; Data Bridge Market Research
[6] Elevator Market Size; Global Market Insights
[7] D. Niu, L. Guo, W. Zhao, H. Li, Operation performance evaluation of elevators based on condition monitoring and combination weighting method. Measurement, 194, 2022.
[8] X. Zhang, M.U. Zubair, Extending the useful life of elevators through appropriate maintenance strategies. Journal of Building Engineering, 51, 2022.
[9] X.-Y. Jiang, X.-C. Huang, J.-P. Huang, Y.-F. Tong, Real-Time intelligent Elevator Monitoring and Diagnosis: Case Studies and Solutions with applications using Artificial Intelligence. Computers and Electrical Engineering, 100, 2022.
[10] D. Niu, L. Guo, X. Bi, D. Wen, Preventive maintenance period decision for elevator parts based on multi-objective optimization method. Journal of Building Engineering, 44, 2021.
[11] S. Junnila, A. Horvath, A.A. Guggemos, Life-Cycle Assessment of Office Buildings in Europe and the United States. Journal of Infrastructure Systems, 12, pp. 10-17, 2006.
[12] ISO, Environmental Management – Life Cycle Assessment – Principles and Framework, Geneva, 2006.
[13] ISO, Environmental Management – Life Cycle Assessment – Requirements and Guidelines, Geneva, 2006.
[14] GaBi; Thinkstep, thinkstep.com. Accessed: 2019.
[15] R. Corner, Project correspondence, 2022.
[16] G. Wernet, C. Bauer, B. Steubing, J. Reinhard, E. Moreno-Ruiz, B. Weidema, The ecoinvent database version 3 (part I): overview and methodology. The International Journal of Life Cycle Assessment, 21, pp. 1218-1230, 2016.
[17] AP 42, Fifth Edition, Volume I Chapter 12.8 Secondary Aluminum Operations; EPA, epa.gov/air-emissions-factors-and-quantification/ap-42-fifth-edition-volume-i-chapter-12-metallurgical-0. Accessed: June 2022.
[18] Aluminium Recycling; ALFED, alfed.org.uk/files/Fact%20sheets/5-aluminium-recycling.pdf. Accessed: June 2022.
[19] Aluminium Recycling Factsheet; IAI, international-aluminium.org/resource/aluminium-recycling-fact-sheet/. Accessed: June 2022.
[20] Recycling rate of electrical and electronic waste in the United Kingdom (U.K.) from 2010 to 2018; Statista, statista.com/statistics/632826/e-waste-recycling-uk/. Accessed: June 2022.
[21] Directive 2012/19/EU of the European Parliament and of the Council of 4 July 2012 on waste electrical and electronic equipment (WEEE) Text with EEA relevance; EU, eur-lex.europa.eu/legal-content/EN/TXT/?uri=celex%3A32012L0019. Accessed: June 2022.
[22] Energy calculator; design.na.tkelevator.com/tools/energy-calculator. Accessed: June 2022.
[23] E. Ozkan, N. Elginoz, F. Germirli Babuna, Life cycle assessment of a printed circuit board manufacturing plant in Turkey. Environ Sci Pollut Res Int, 25, pp. 26801-26808, 2018.
[24] ReCiPe 2016 – A harmonized life cycle impact assessment method at midpoint and endpoint level – Report I: Characterization; M.A.J. Huijbregts, Z.J.N. Steinmann, P.M.F. Elshout, G. Stam, M.D.M. Vieira, A. Hollander, M. Zijp, R. van Zelm, rivm.nl/bibliotheek/rapporten/2016-0104.pdf. Accessed: June 2022.
Get more of Elevator World. Sign up for our free e-newsletter.