Energy Efficiency of Elevators
May 1, 2025
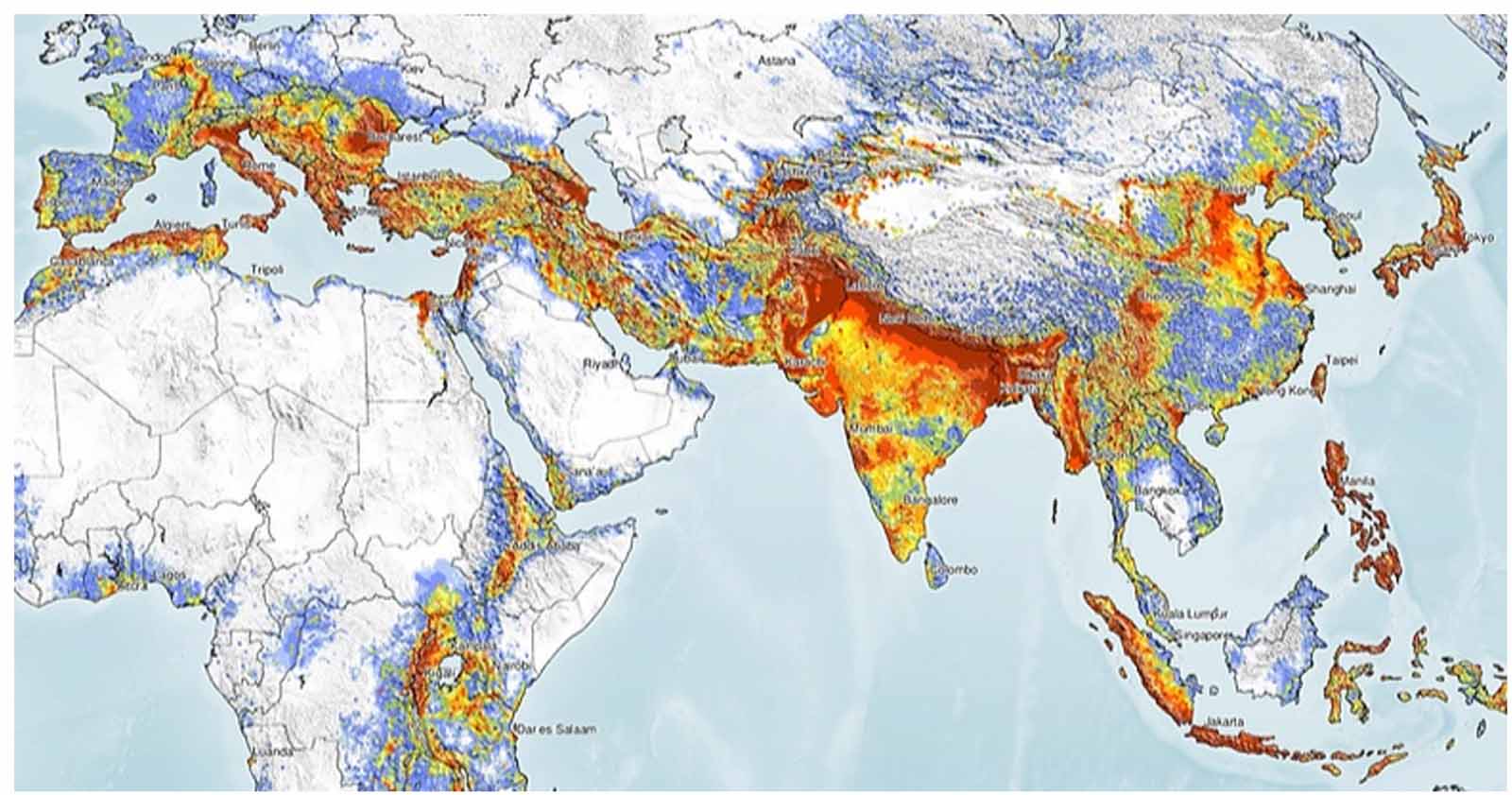
MRL vs. hydraulic
by Dr. K. Ferhat Çelik
1. Introduction
Elevators are complex engineering systems specifically designed and installed for individual building shafts. Depending on their usage, they account for approximately 2% to 10% of a building’s energy consumption. For low-usage elevators, such as buildings with up to five floors, this percentage typically ranges from 2% to 3%. Overall, elevator energy consumption is relatively low compared to a building’s total energy demand, which often exceeds 40% of the total usage. Consequently, for example, when evaluating a building’s energy performance, the primary focus should be on thermal insulation, heating and cooling systems, hot water and lighting. Similarly, addressing operational elevator energy consumption alone is insufficient to fully understand an elevator’s total energy performance and can lead to inaccurate conclusions. Such an approach also risks prioritizing elevators wrongly based on incomplete data, potentially overlooking critical safety factors.
Various theoretical and hybrid calculation methods have been developed to classify elevators in terms of their energy performance and consumption. Standards like VDI 4707-1 and ISO 25745/1-2 initially encouraged elevator companies to leverage these classifications as marketing tools, particularly in the low-rise market. However, elevators marked with a class “A” energy label may actually achieve significantly lower performance when assessed under realistic conditions. Accurate evaluation of elevator energy performance requires reliable data, including the number and intensity of trips, travel speed, loading conditions, stand-by energy consumption and counterweight load, all of which are influenced by elevator traffic patterns. Today, those manipulative classifications have lost their importance and are replaced by Life Cycle Analysis (LCA).
Although the reliability of such calculation models is questionable, an unfortunate perception has emerged in the market that the energy consumption ratios of elevators are approximately 3:2:1 for hydraulic, geared traction and gearless machine-room-less (MRL) systems, respectively. This paper provides detailed information about elevator energy performance based on realistic evaluation.
2. Safety Against Earthquakes
Elevators are among the most expensive equipment in buildings and serve a crucial function. However, they contain various mechanical and electrical/electronic components that are particularly vulnerable to damage during natural disasters, especially earthquakes.
Europe and Asia are highly prone to earthquakes; therefore, ensuring that elevators remain operational after seismic events is crucial for minimizing related risks. The EN 81-77 standard outlines additional measures to enhance elevator safety in seismic regions. However, implementing this standard alone does not guarantee complete safety in earthquake-prone areas. This was also observed in field inspections conducted after the February 6, 2024, earthquakes in Türkiye. Although the EN 81-77 standard aims to ensure the safe and secure use of elevators in earthquake-prone areas, it falls short in implementing effective measures to prevent the drawbacks of traction elevators with counterweights, particularly their machine-room-less designs, which present numerous safety-related issues. Enhanced safety can only be achieved by making the necessary additions to the standard, at the cost of increased expense. Therefore, the national standardization office in Iran should incorporate additional requirements when implementing IR EN 81-77.
While shortages in the EN 81-77 standard and its implementation are often cited as primary causes of damage, the main issue is the failure to select the appropriate elevator type based on building characteristics. In seismic regions, apart from assessing the risk of elevator damage, it is equally important to consider emergency evacuation. Factors such as fire hazards, gas leaks and flooding should be accounted for to ensure the safe rescue of trapped passengers. Beyond repair costs resulting from elevator damage, downtime can lead to significant social and commercial losses. In a post-earthquake environment marked by social trauma and ongoing aftershocks, keeping public buildings and their elevators operational is essential for effective emergency response management. Therefore, to minimize the challenges after earthquakes, the first priority should be given to elevator systems with low damage risk that can be easily and cost-effectively adapted to comply with IR EN 81-77 standards. Reports from field inspections after several earthquakes have already made it clear that hydraulic elevators are the safest choice for seismic regions. This is because:
- The hydraulic cylinder absorbs vibrations and oscillations, reducing seismic impact.
- Hydraulic elevators do not have counterweights, eliminating related damage risks.
- Hydraulic systems rest directly on the building foundation; therefore, they experience smaller oscillations and are less affected by seismic movement.
- They have a simple structure with fewer components, which makes them more reliable.
- They provide easier and safer evacuation in case of entrapment.
In contrast, the most vulnerable and unsafe elevator type for seismic regions is the MRL type. Further discussions on this topic can be found in references.[10] Some examples of elevator damage after an earthquake are shown in Figure 2.
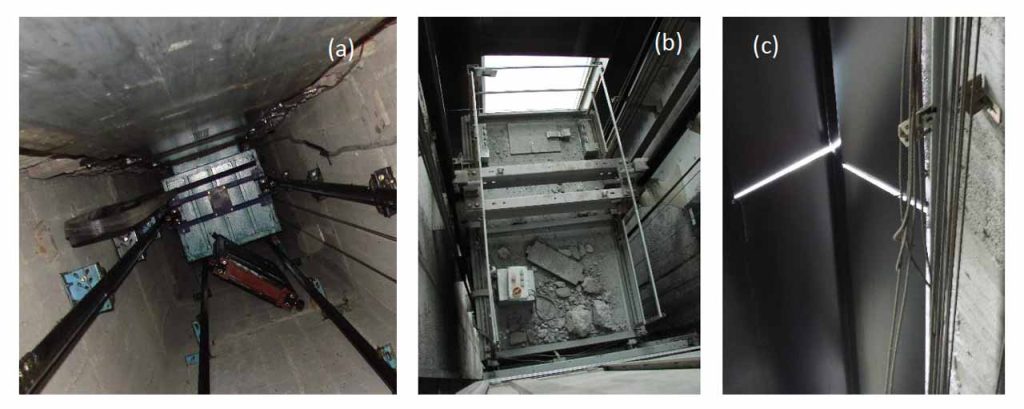
3. Stand-By Energy Consumption
As a result of advancements in electronic control systems, energy utilization has increased not only during elevator operation but also while the system is idle. The energy consumed by control systems in idle mode is referred to as “stand-by energy consumption.” The incorporation of features such as uninterruptible power supply (UPS) devices, door-lock systems, drives, illuminated push buttons, cabin displays (internal and external), warning and safety systems and other similar electronic components has significantly increased the amount of stand-by power required to keep these systems active.
With the introduction of MRL elevators, variable speed drives began to be widely adopted in traction elevators. These drives, combined with permanent magnet synchronous (PMS) motors, enhanced riding quality and reportedly reduced operational energy consumption by up to 50% compared to conventional traction elevators by employing smaller motors.[1]
In addition to MRLs, variable-voltage, variable-frequency (VVVF) drives have also been implemented in conventional traction elevators equipped with machine rooms and gear systems. In response to this, hydraulic elevator solutions featuring VVVF drives have entered the market as competitive alternatives, intensifying competition in the low-rise building segment. Consequently, the power drawn by these drives and their associated peripheral devices has become a notable contributor to overall elevator energy consumption.
A study conducted by the Swiss Agency for Energy Efficiency (SAFE)[2] on 33 different elevators reported that standby mode energy consumption accounts for approximately 80% of the total energy used by elevators. Figure 3 illustrates the significant impact of standby mode energy consumption (orange), which has risen due to the widespread incorporation of electronic components in elevator systems. The study also highlights that hydraulic solutions equipped with VVVF drives are as energy-efficient as traction MRL systems. This is because the energy losses associated with conventional mechanical control valves are completely eliminated through the use of VVVF drives in hydraulic elevators.
An MSc thesis by Lees,[3] which examined the energy consumption of hydraulic elevators, concluded that hydraulic systems using VVVF drives are as energy-efficient as MRL systems. Studies on Blain’s EV40-VVVF hydraulic control valve also demonstrated up to 65% energy savings, making it a popular choice for modernization projects.[7] These findings challenge the general perception that hydraulic elevators consume more energy than traction MRLs. However, Lees also noted that in low-usage elevators, the use of VVVF drives could increase stand-by energy consumption. As a result, conventional hydraulic elevators with mechanical valves remain a viable option in such cases. This suggests that despite advancements in elevator technology, energy consumption can still increase in certain scenarios, particularly for low-usage systems.
Energy-efficient systems are expected to repay their investment over time through reduced energy bills. The speed of this payback depends on various factors, including the cost of energy, elevator usage, system efficiency, initial investment cost and the frequency and expense of maintenance. Highly efficient systems often incorporate regenerative braking, which feeds electricity back into the building instead of dissipating it as heat. While this technology is effective, it requires a significant upfront investment, making it viable primarily for elevators with very high usage. For low-to-mid-usage elevators, incorporating a regenerative unit (regen drives) will be environmentally and economically impractical, as the investment is unlikely to be recovered within the system’s service life. In such cases, the resources and energy used to manufacture and install energy-efficient equipment may have a greater environmental impact than the benefits gained from reduced energy consumption. Thus, if an investment is not cost-effective, it is unlikely to achieve true energy efficiency.
Elevator control systems designed for optimal energy use during stand-by periods are also available in the market. In these systems, energy consumption is gradually reduced when the elevator is idle for a certain duration. In the first stage, car lights are dimmed and direction indicators on floors, car displays and dual illuminated buttons are turned off. In the second stage, additional components such as the door controller, cabin electronics, inverter fans and dual-illuminated buttons on floors are powered down. Reactivating the system from this mode typically takes about 30 s. However, the primary sources of standby energy consumption are the VVVF drive and the UPS device, which are generally not powered down to avoid reducing the lifespan of the drive and for safety reasons. Tables 1, 2 and 3 show the energy consumption of the elevator controller, drives and UPS devices.
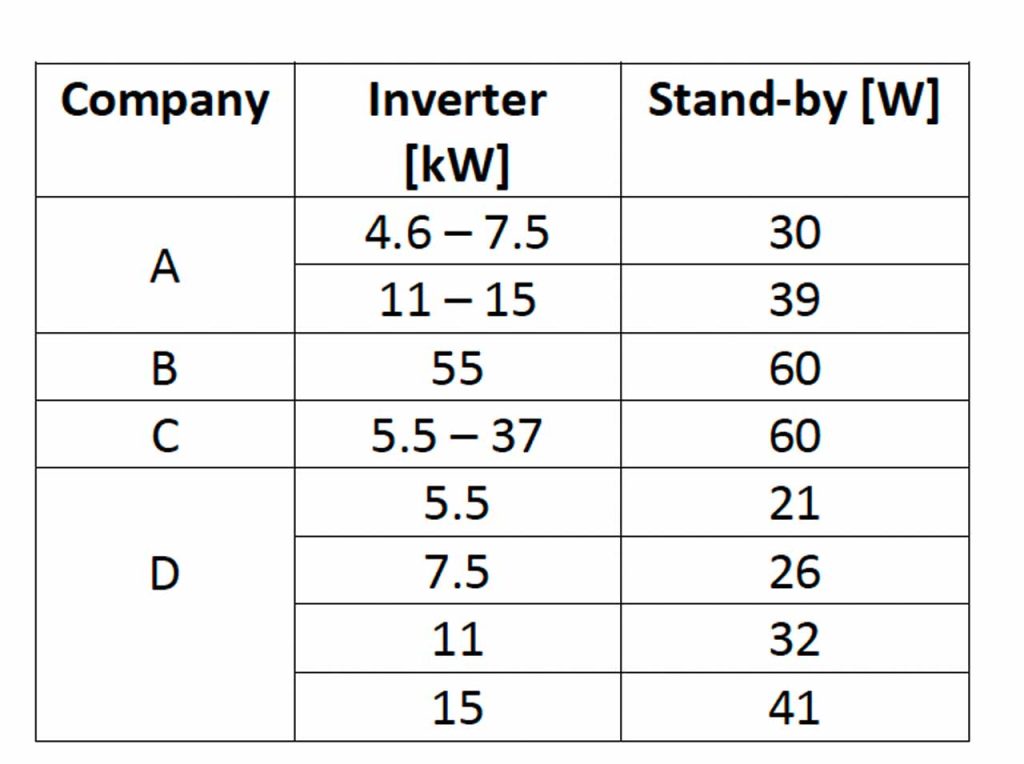
4. Which Type of Elevator Is Best for Saving Energy?
All elevators share common components, such as cars, doors, lights, ventilating fans, safety devices and automatic controls. Since these elements are standard in both traction and hydraulic elevators, this discussion focuses specifically on the energy efficiency of drive systems.
More than 90% of the global building stock consists of buildings with fewer than six floors. Therefore, the main competition on the market is actually where both hydraulic and traction elevators can be utilized. MRL elevator systems, initially introduced in 1995 by large elevator companies, are now widely offered by most elevator manufacturers. PMS gearless traction machines, delivering high torque and low speed while eliminating the need for reduction gears, significantly improved system efficiency. The PMS machines’ compact size, reduced weight and unique dynamic features — such as high stability, precision, stronger torque at low speeds and accurate rotor position control — have made them ideal for elevator drive systems. These advancements paved the way for the development of MRL elevators. MRLs, where the drive unit is placed inside the shaft, are utilized for low- to mid-rise elevator installations, and they are increasing in popularity.
The introduction of MRL elevators has clearly improved the operational energy efficiency and control characteristics of traction drives, enabling their installation in low-rise buildings. However, several critical issues have been overlooked in the process of MRLs being enthusiastically embraced by the elevator industry.
Elevator Safety
Safety is the top priority in elevator applications. MRLs are known as the least safe elevators on the market. Leaving secure machine rooms to placing the drive system in the headroom represents a step backward in safety for the elevator industry. Therefore, MRLs require additional components to meet safety standards under the less secure working conditions. The increase in component complexity raises the risk of failures during installation, servicing and maintenance. As MRL applications become more widespread, reports of MRL-related accidents have increased globally. Safety codes for MRLs remain questionable and require stricter regulations and revisions. However, these advancements often depend on the influence of large elevator companies, which prioritize cost reduction over implementing additional safety features in such systems. Notably, MRLs have proven to be the least durable elevators in seismic regions, as documented by site investigations following various earthquakes. Countries with high earthquake risks, such as Türkiye, Iran and a major part of Asia, should prioritize safety over energy consumption and avoid using MRL in seismic regions. This is particularly important given that elevators are not a primary contributor to energy consumption issues. For this reason, elevators are exempt from the Eco-Design Directive in the European Union.
On the other hand, hydraulic elevators are the most durable and safest among all types of elevators. Their secure machine room can be conveniently located in the basement or on the ground floor near the elevator shaft, allowing for safe and efficient installation. With all components operating in a lubricated environment, hydraulic elevators boast high reliability with a service life exceeding 30 years. They are also the most resilient during earthquakes, as their hydraulic cylinders are anchored to the building’s foundation. Most importantly, the cylinder functions as a damper, protecting the entire elevator system from damage. Numerous post-earthquake site investigations, including the recent one in Türkiye, have consistently demonstrated the robustness of hydraulic elevators in seismic regions.
Although hydraulic elevators have energy-efficient control valves compatible with MRLs, the key question is why their global usage remains significantly lower than that of MRLs. The primary reason is the dominance of large elevator companies promoting MRL solutions, while hydraulic companies are relatively small in size. This limited presence has hindered the hydraulic sector’s ability to compete and protect its market share.
Secondly, as hydraulic elevators have lost market share, industry expertise in hydraulic systems has declined. This reduction in knowledge has contributed to hydraulic elevator quotes becoming more expensive than MRL solutions, despite their potential to be more energy-efficient and/or safer. The lack of expertise in hydraulic design and setup has led to an increase in poorly executed and low-quality hydraulic elevator installations, further damaging the reputation of hydraulic systems and reducing their demand in the market.
Finally, the global elevator market is largely driven by major elevator companies, which frequently adopt aggressive low-price policies to secure projects. These companies offset lower initial costs with revenue from long-term service contracts, further disadvantaging smaller players in the hydraulic sector.
Total Energy Consumption
Focusing solely on operational energy consumption of mechanical valves as well as oil usage, and using those issues as a marketing tool is a rather questionable approach promoted by large elevator companies to capture the low-rise market. As a result, the MRL solution has been portrayed as always offering the most energy-efficient option, perfectly suitable for every installation and capable of regenerating energy to be fed back into the grid. However, the claimed benefits of MRLs are not always factual and may lead to higher energy consumption, particularly in low-usage elevators[4] where the investment in MRL technology may never be gained back during the elevator’s lifespan.[5] This is because MRL controllers require more electronic and electrical components — such as drives, drive cooling fans, UPS devices, safety circuits and other peripheral devices — which are costly and consume additional energy even when the elevator is at rest.[1] Therefore, the energy consumption of elevators is directly related to their usage.
Before selecting an MRL system, the daily energy consumption of the elevator controller should be compared with the expected usage patterns. Additionally, to reduce costs, many MRL drive systems now utilize asynchronous motors with gearboxes instead of the more expensive PMS gearless motors. However, as the gear ratio increases, system efficiency decreases due to significant power losses in the gear mechanism. These systems fall short in delivering the substantial energy savings and high performance that PMS motors provide.
Low-Usage Elevators
MRLs are not energy-efficient for home elevators or those used for special purposes with fewer than 100 starts per day —potentially this can expand to up to 300 starts per day, depending on the controller efficiency of the MRL system. For such applications, hydraulic elevators with mechanical valves are the better choice, not only because of their lower energy consumption but also due to their superior safety features and ease of installation. In low-usage setups, the standby energy consumption of MRLs often exceeds that of hydraulic elevators using mechanical valves. Additionally, the lower reliability of MRLs compared to hydraulic systems makes them less suitable, particularly when considering the risk of entrapments. Hydraulic elevators, on the other hand, can provide a self-rescue mechanism inside the car, a critical safety feature for villas and elevators designed for elderly users.
Medium- and High-Usage Elevators
Elevators performing between 200 and 500 starts per day can be classified as mid-usage. For such usage levels, both MRL systems and VVVF-driven hydraulic systems (such as the Blain EV40-VVVF) offer comparable energy consumption performance. However, the lifespan of the drive is influenced by the number of starts. Hydraulic solutions, which utilize the drive only in the upward direction, maximize energy savings and effectively double the drive’s lifespan compared to MRL systems. Alternatively, servo electronic valves (such as Blain’s SEV07) can also be used in this category, depending on energy performance requirements.[6] Electronic valves, which can save up to 30% energy, are particularly effective in the transition region between low- and high-usage categories. Since electronic valves do not require a VVVF drive and its associated peripheral devices, they offer lower standby energy consumption and reduced energy losses.
Elevators performing more than 500 starts per day fall into the high-usage category, where both MRL systems and VVVF-driven control valves are viable options. In this category, though VVVF-driven hydraulic systems can reduce heat generation by up to 50%, proper air ventilation in the machine room remains necessary. For systems exceeding 1,100 starts per day, additional measures, such as a small-capacity oil cooler, may be required alongside adequate ventilation to ensure optimal performance for VVVF-driven hydraulic solutions. In such extremely high usages, traction elevators can be preferred when energy savings are the primary goal. However, for applications prioritizing safety and reliability, such as in seismic regions or where breakdown-free operation is critical, hydraulic elevators remain the superior choice.
It is expected that future developments in drive technology will reduce stand-by energy consumption and cost of the drives. In this case, properties of environmentally suitable solutions that are safer, simple, inexpensive, easy to maintain and offer high compatibility with existing systems are expected to find wide applications in coming years. Following this perspective, MRLs require the most intensive service, are the least safe and are difficult to install and maintain. This is because they are installed in the headroom and possess more components to provide sufficient safety, which cannot be guaranteed under unsafe installation and service conditions. In contrast, hydraulic elevators have the safest and most durable characteristics against breakdown, installation and maintenance in the low-rise market.
5. Use of Counterweight With Hydraulic Elevators
It is well known that reduced energy consumption of the traction elevator results from the counterweight. The hydraulic elevator generally does not use counterweights and is consequently simpler to install and is less costly and safer (particularly in seismic areas) at the expense of increase in motor power. To decrease the motor kilowatt size, it is important to have lighter car and car frame. Use of composite materials for the car and the frame enables cars to be produced about 70% lighter. Although the initial cost of composite manufacturing at present is high, integrating the car and the frame will simplify the installation, and using smaller size motors reduce energy consumption considerably.
On the other hand, though it is not preferred, hydraulic elevators can be constructed with the counterweight under suitable conditions (in earthquake-free regions) so that a good amount of energy savings can be obtained. Table 4 shows the motor power of a hydraulic elevator for eight people with and without a counterweight. It can be seen that by reducing 2/3 of the car weight (by means of a counterweight) the motor power can be reduced by 29%.
In Figure 4 another configuration of the counterweight for the hydraulic elevators is shown, where the counterweight is placed on a pull ram. By doing so, smaller diameter rams and pumps can be used. Such a configuration can reduce motor power and pump flow rate by up to 40%. The design also prevents the counterweight from swinging in the shaft.
6. Comparison of Environmental Impact by LCA
LCA is a methodology used to assess the environmental impact of products. In the case of elevators, LCA provides a comprehensive means of evaluating sustainability by considering total energy/impact performance throughout their entire lifespan, from raw material extraction to disposal. The LCA approach offers a more accurate representation of an elevator’s environmental footprint. In contrast, focusing solely on energy consumption of elevators and assigning energy performance classes are marketing strategies often employed by major MRL manufacturers and should not be highly valued. The real energy performance of elevators can only be determined by measurements at installations.
Hydraulic elevators have a bigger motor and no counterweight. In return, it spends no energy in the down direction, and descends with its own weight. Having no counterweight makes the elevators simpler to install with high reliability and is safer and cost effective, which are added values for the LCA.
The results of an LCA study for four-stop elevators operating at a capacity of 450 kg, with usage categories of 1, 2 and 3 (ranging from 50 to 300 starts per day), were reported by ITAINNOVA, the Technological Institute of Aragon,[8] as shown in Figure 5. These results compare a traction MRL elevator and a conventional hydraulic elevator. It should be noted that although MRL elevators require corrective maintenance more frequently, the “product category rules for lifts” procedure, which outlines how to apply LCA to elevators, only considers periodic maintenance and does not account for the corrective maintenance (additional repairs needed when problems arise). In other words, if the corrective maintenance had been included in the LCA, the results would have been more unfavorable for the MRL elevator. According to the Ecoindicator ReCiPe method, the environmental impact results for hydraulic elevators are consistently lower over a lifespan of 22 years (Figure 6).
In the case of modernization, hydraulic lifts always have less impact on the environment than any other new MRL traction lift. This is because most of the components of the existing hydraulic system can be reused, such as the cylinder, tank, connectors, etc. for modernizations. In actual fact, modernizing a hydraulic lift with a traction MRL results in worse impact to the environment (see Figures 5 and 6). In the LCA, when 80 years of building lifespan is used with an assumption that the elevator is modernized every 20 years, then the hydraulic elevator also becomes environmentally more preferable even with the usage category 3 (300 starts/day).[9]
In general, hydraulic elevators are much more reliable than traction elevators, which means their maintenance has a much lower impact on the LCA. Their simple design, with fewer components and a fully lubricated system, helps extend their lifespan and reduce system downtime, leading to significant cost savings. It is important to note that elevator maintenance costs can be two to 10 times higher than the yearly energy consumption. Additionally, the most expensive component of a traction MRL drive system makes up over 30% of the total elevator cost, while for hydraulic elevators, it is only about 6% to 8%. Based on these findings, conventional hydraulic elevators with a mechanical control valve in low-rise buildings (with up to 300 starts per day) have a lower overall environmental impact, including total energy consumption, compared to traction MRL elevators.
7. Selection of the Hydraulic Control Valve
There are mechanical, electronic and VVVF-driven elevator control valves available in the market. Among these, VVVF-driven valves are the most energy-efficient, capable of reducing energy consumption by up to 65% and heat generation by 50% for mid- and high-usages.[7] Despite their lower efficiency, mechanical control valves are the most widely used. This is because they are currently the most suitable option for low usage applications (for home and residential elevators), due to their significantly lower cost, simple adjustments and high reliability. Furthermore, the payback period for VVVF-driven valves is rather long for applications with fewer than 350 starts per day. However, some hybrid VVVF-driven control valve solutions, such as the Blain’s Smart EV40-vvvf, are available at reasonable prices and offer payback periods of two to four years for elevators with more than 200 starts per day.[7] These hybrid solutions use the VVVF drive only during upward travel, which makes them the most energy efficient option for low-usage elevators. Additionally, when an energy-efficient drive such as the Yaskawa GA700 is used and shut down after 10 min of idle time, hybrid VVVF solutions can be implemented for lower usage levels of 130 starts per day. Hybrid VVVF-driven control valves with an energy-efficient drive appear to be the ideal choice for low-usage elevator applications. It is important to note that regenerative drives are generally unsuitable for low-usage applications due to unacceptably long payback periods, as they recover minimal energy while incurring high costs.
8. The SMART EV40-VVVF
Blain Hydraulics made use of Internet of Things (IoT) technology and embedded systems to collect, send and act on data collected from the environment and evolved the previously designed EV4-VVVF product into the Smart-EV40-VVVF solution (Figure 5). The Smart-EV40-vvvf is a hybrid system that offers the following advantages:
- Uses a standard GA700 Yaskawa drive
- Provides high ride quality
- Offers easy diagnostics via an interface board
- Provides up to 65% energy savings and 50% heat reduction
- Lift operation can be monitored, recorded and adjusted by a smart device using Wi-Fi connectivity.
- Boasts easy wiring with the drive and the lift controller
- Features input parameters that have been reduced by 60%.
- No additional pressure switches are necessary.
- By-pass and leveling times are reduced, which further improves energy savings.
- Is delivered with an integrated unintended car movement safety valve.
Figure 7 and Figure 8 show the connections Smart-EV40-VVVF solution and some input menus of the application software, respectively.
9. Conclusions
Standby energy consumption and usage patterns must be carefully considered to achieve optimal energy efficiency in elevators. While traction MRL elevators with VVVF drives have been introduced as energy-efficient solutions, hydraulic elevators equipped with VVVF drives have also gained market presence, offering comparable energy efficiency. However, the efficiency of these systems diminishes with low usage, where simpler elevators with significantly lower stand-by energy requirements become more advantageous. Therefore, for low-usage home and residential elevators, hydraulic elevators with mechanical valves remain a superior choice. They offer low energy demand, affordability, easy setup, simple maintenance and high reliability, making them an ideal solution in such applications.
Blain’s Smart-EV40-VVVF solution employs IoT and advancements in digital technology to address system weaknesses and enhance usability. With a user-friendly mobile app, the system setup can be completed quickly, while lift operation can be monitored, recorded and adjusted using a smart device connected via Wi-Fi. In addition to delivering a smooth and superior ride quality, the Smart-EV40-VVVF offers shorter bypass and re-leveling times, reducing both total travel time and energy consumption.
It is important to emphasize that in elevator applications, safety must take precedence over energy performance. In earthquake-prone areas, for high-rise buildings, traction elevators with machine rooms should be installed when necessary. MRL systems should be avoided unless additional safety measures are implemented. For low-rise buildings in seismic regions, hydraulic elevators should be the first choice due to their superior durability and damping properties, which effectively mitigate the impact of seismic shakes. As the energy consumption of elevators in low-rise buildings is not very significant, it is better to prioritize seismically safe elevator systems over energy efficiency. This approach ensures that buildings remain operational, facilitates easy and safe passenger evacuation, and helps prevent social, commercial and human losses.
References
[1] H. M. Sachs, “Opportunities for elevator energy efficiency improvements,” American Council for an Energy Efficient Economy, April 2005.
[2] J. Nipkow, „Elektrizitätsverbrauch und Einspar-Potenziale beä Aufzügen,“ S.A.F.E., 2005, Zürich.
[3] G. Lees, “A study of the actual power relative to the theoretical power consumption of a variable frequency drive hydraulic system and how it benefits the user,” Master dissertation, University College Northampton, April 2005.
[4] Almedia A.T., Energy Efficiency of Elevators & Escalators, 4th European Lift Congress, 2010.
[5] Celik K.F., “Stand-by Energy Consumption on Low Usage Lifts,” ELEVATOR WORLD India, Vol.2, pp.58, 2009.
[6] Celik K.F., “Design and Control of Electronic Control Valves,” Elevator Technology 17, Proc. Of Elevcon, pp.34-45, 2008
[7] Celik K.F., “A Load & Temperature Compensation Method for Green Hydraulic Lifts by means of Inverters,” Proceedings of Elevcon, IAEE, Miami, 2012.
[8] ITAINNOVA, Instituto Technologico de Aragon, Life Cycle Assessment for Hydraulic and Traction Lifts, October 2017.
[9] hydroware.de/lib/get/file.php?id=155f9221554af7
[10] F. Celik, “Elevator safety in seismic regions,” EW, August 2005, p.140.
Get more of Elevator World. Sign up for our free e-newsletter.