Voltage Sags
Feb 1, 2015
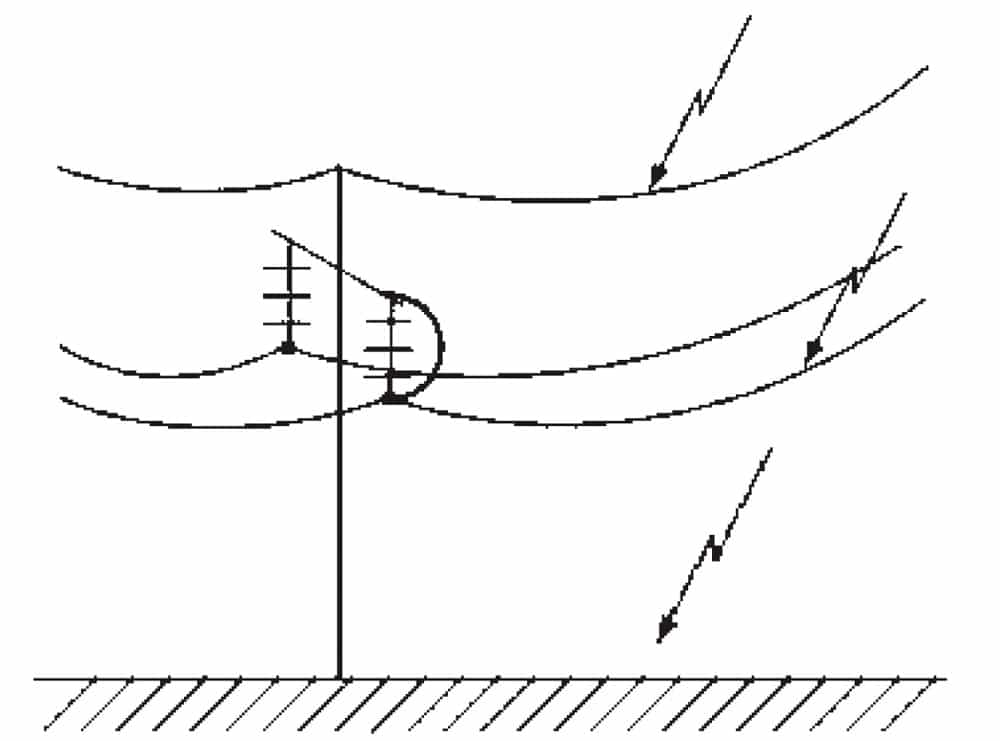
How these phenomena affect the elevator industry and steps that can be taken to mitigate their effects
by Dr. Albert So and Dr. W.L. Chan
Your authors were first inspired to look into the issue of voltage sags in 2004 after a serious sag due to a heavy thunderstorm in Hong Kong caused more than 50 elevators to halt, trapping quite a number of passengers. Normally, when an elevator fails to operate and its passengers are trapped, building management calls the maintenance contractor first. And, if there is any potential passenger injury, help from the police, then firefighters is sought. This practice is, perhaps, usual anywhere around the world. But, if we think about a situation in which hundreds of elevators are tripped at the same time due to an electric power problem (voltage sags and blackouts being two possible causes to consider), is it likely that adequate support from both maintenance contractors and firemen is available to rescue thousands of trapped passengers? Furthermore, after a series of voltage sags followed by immediate elevator stoppages somewhere along the hoistway in Hong Kong from 2004 to 2006, debate began on who should bear the responsibility: the electric utility, elevator manufacturer and/or maintenance contractor?
Learning Objectives
After reading this article, you should:
♦ Understand why electric power quality has become a major issue
♦ Know the definition of and attributes by which to assess the electric power quality of a system
♦ Have more knowledge on voltage sags
♦ Understand the effect of voltage sags on elevator systems
♦ Have an idea of various solutions to mitigate the effect of voltage sags on elevator systems
Power quality issues are of increasing concern, because devices such as computerized controls, variable-speed drives, automated intelligent smart devices and sensors are sensitive to fluctuation in supply voltage. Users and manufacturers are usually not aware of the impact of voltage sags when they purchase or produce new equipment. Once the equipment is in operation and suffers from stoppage due to voltage sags, the utility is usually criticized for poor power quality. This is the reason why users demand zero voltage fluctuations from a utility, rather than expecting the manufacturer to provide electrical equipment with appropriate power-quality compatibility.
Electric Power Quality and Voltage Sags
Voltage sags caused by adverse weather conditions could be unavoidable. The term “voltage sag” is used by the trade in the U.S., while “voltage dip” is widely used in Europe. One of the best references to the issue of electric power quality is the Institute of Electrical and Electronics Engineers (IEEE) Standard 1159-2009 on the recommended practice for monitoring electric power quality. According to the standard, the term “power quality” refers to a wide variety of electromagnetic phenomena that characterize the voltage and current at a given time and at a given location on the power system. The recent increase in attention to electric power quality has been due to the popularity of active (nonlinear) power electronic devices, such as rectifiers or choppers of high power rating in which the three phases are not equally consumed and/or the current waveform is no longer sinusoidal. The standard makes use of an electromagnetic compatibility (EMC) approach to describe power-quality phenomena.
According to the International Electro-technical Commission (IEC), EMC describes the ability of electronic and electrical systems or components to work correctly when they are close together. In practice, this means that the electromagnetic disturbances to others from each item of equipment must be limited and that each item must have an adequate level of immunity to the disturbances in its environment. The ultimate target is to make sure all equipment can enjoy a high level of reliability and safety when working together with other equipment.
IEC classifies the principal phenomena that cause electromagnetic disturbances into groups. The “conducted low-frequency phenomena” group is related to harmonics, voltage fluctuations, voltage sags and interruptions, voltage imbalance, power-frequency variations, induced low-frequency voltages, and the existence of DC components in an AC network. While this group usually concerns the elevator industry the most, there are other groups like “radiated low-frequency phenomena” related to magnetic and electric fields, and “conducted high-frequency phenomena” related to induced continuous-wave voltages or currents, unidirectional transients, oscillatory transients, etc.
Such phenomena could be assessed for a steady-state study in accordance with IEC 61000-2-5 by attributes including amplitude, frequency, spectrum, modulation, source impedance, notch depth and notch area. For a non-steady-state study, alternative attributes, such as rate of rise, duration, rate of occurrence and energy potential, are involved.
For example, in the standard, to describe a category of “long duration root-mean-square (rms) variations,” the typical duration of an interruption, undervoltage, overvoltage or current overload is longer than 1 min., while the amplitude of interruption is 0.0 per unit (pu), that of undervoltage is 0.8-0.9 pu, and that of overvoltage is 1.1-1.2 pu. Here, 1.0 pu, being a dimensionless quantity, refers to 100% of the rate value. The duration of power-frequency variations is usually less than 10 s. long, while the amplitude of variation is ±0.1 Hz.
Now, we come to voltage sags. A voltage sag refers to a short duration, usually up to 1 min., when the magnitude of voltage supplied to a system is reduced. The term “swell” is an inverse to sag. In the standard, a category known as “short-duration rms variations” is used to include sags, swells and interruptions. Instantaneous sags (a magnitude from 0.1 to 0.9 pu) and swells (a magnitude from 1.1 to 1.8 pu) have a duration of 0.5-30 cycles (i.e., 0.0083 to 0.5 s. in a 60-Hz system). Momentary interruptions (a magnitude less than 0.1 pu) have a duration from 30 cycles (0.5 s.) to 3 s. Momentary sags (a magnitude from 0.1 to 0.9 pu) and swells (a magnitude from 1.1 to 1.4 pu) have durations from 30 cycles (0.5 s.) to 3 s. Temporary interruptions (a magnitude less than 0.1 pu), sags (a magnitude from 0.1 to 0.9 pu) and swells (a magnitude from 1.1 to 1.2 pu) have durations from longer than 3 s. to 1 min.
Voltage sag is only one issue within the whole family of electric-power-quality problems, which also includes:
- Sudden but very short increases in voltage, which are usually called “spikes,” “impulses” or “surges”
- Undervoltages, sometimes called “brownouts,” that cause dimming of incandescent lamp bulbs (different from “blackouts” [no voltage])
- Harmonics, i.e., the existence of high-frequency components bearing frequencies that are multiples of the fundamental, such as 50 Hz or 60 Hz, that may lead to interference and low energy efficiency
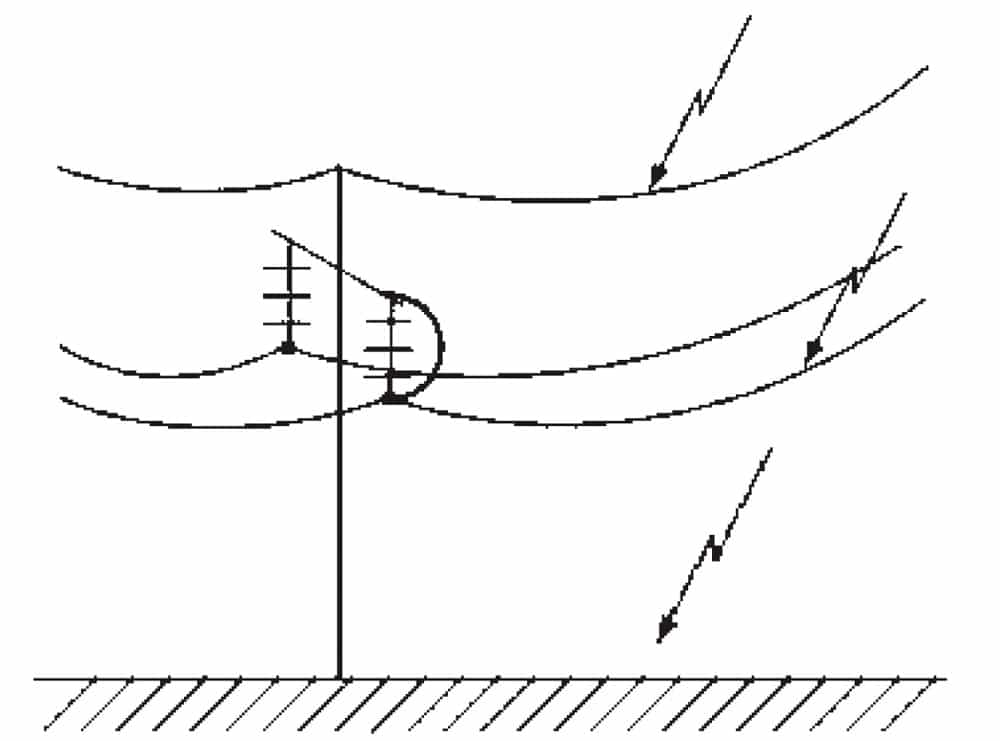
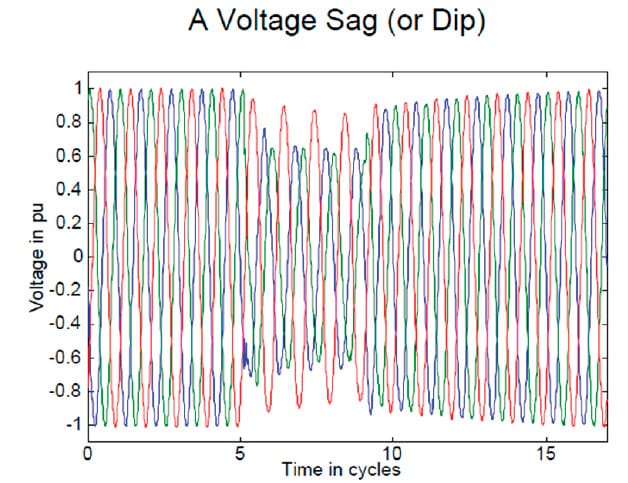
A voltage sag is not considered a power failure or interruption, which often only lasts up to 0.2 s., after which normal power supply will be restored. (Note that the formal definition, however, allows a prolonged existence up to 0.5 s. for an instantaneous sag.) There could be many causes of such an event, most of which are associated with bad weather conditions. During a thunderstorm, lightning strikes often hit the overhead transmission lines responsible for the transmission of electric power from the generating plant to substations. Such overhead lines are usually found in the suburbs, across mountain ridges or on plains, where they are the tallest nearby objects. Although the tip of transmission towers is connected by an earthed shielded or guarded wire for protection, while the transmission voltage (say, at 400-800 kV) is a bit closer to the voltage of the lightning strikes, there are still chances of the overhead lines being hit by them.
After hitting a phase conductor, the lightning strike continues its way to the ground, either via the tower itself or immediately downward (Figure 1). In this way, a short voltage sag is caused (Figure 2), because the path of the lightning strike from the phase conductor to the ground is virtually an intermittent short-circuit. In addition to third-party interference due to engineering projects, external interferences, such as plants, animals and flying objects could also cause voltage sags.
At the same time, overvoltages originated by lightning are major causes of flashovers on overhead power lines. These may cause permanent or short interruptions, as well as voltage sags on distribution networks. Additionally, lightning-originated surges can also damage the power components and electronic devices connected to these networks.
Risks of Voltage Sags or Blackouts
When your authors investigated the aforementioned incidents in Hong Kong, the first stage involved a brainstorming session with representatives from the local elevator industry. Afterward, actual site tests with an artificial voltage-sag generator were conducted. Finally, some proposed solutions were tested onsite.
It was found that most practical voltage sags are within a period of 0.2 s. Their impact could be on the elevator controllers, power electronic drives, doors and safety circuits. Out of these, the motor drives are mostly susceptible. This is because, upon a sudden voltage sag, the drive could automatically boost the transient current supplied to the motor, thus probably damaging the electronic circuitry of the drive. Even if the circuitry remains healthy, there is still a chance the drive and/or controller may be tripped due to current overload. Once tripping occurs, the brake would be applied, and the elevator car would stop somewhere along the hoistway with passengers trapped inside. (Here, “tripping” refers to the electric circuit and has nothing to do with the safety devices mandatorily listed in the safety codes.) There would then be the question of whether these electronic devices could be cold booted automatically. If yes, the car could resume normal operation after a while and bring the passengers to a safety stop.
It was found that during the tripping, some controllers lost record of the current position of the elevator car. After restoration to normal operation, the car would be driven slowly to search for some position indicator along the hoistway. If nothing could be found within 45-60 s., the whole system would be tripped again, resulting in passenger entrapment.
There are several concerns here, from the passengers’ point of view. First, the car experiences sudden braking without any smooth deceleration stage right after the tripping, possibly resulting in personal injury. Second, whether the controller could be reset to send the passengers to a safe landing is still unknown. According to ASME 17.1 and EN 81-1, during emergency braking, under a fully loaded down condition, the deceleration upon braking must not be more than 1 g (9.8 mps2/32 fps2). To a normal and healthy person, such deceleration is not critical, though normal braking deceleration is well below that value. However, to an elderly or unhealthy passenger, his or her weight being suddenly doubled when traveling downward may cause a fall, leading to injury. Even if the car is moving upward, the reaction on the soles of passengers’ feet from the car floor is suddenly reduced significantly during emergency deceleration, which can also cause a fall. Although an elevator emergency braking action is still considered safe, it is totally undesirable.
Another concern is related to passenger trapping. If the controller could not be cold booted or reset to work again, or cannot drive the car to update its current position within 45-60 s. under maintenance speed, the car could continue to stop (or stop again), with passengers needing to wait for rescue. Passenger entrapment, though still considered safe in the elevator industry, can jeopardize passengers, whether due to their age, poor health or propensity to self evacuate. Some passengers may start to feel uneasy after being trapped in an enclosed space for as short as 10 min. This concern is not only a possible result of voltage sag, but a certain result of a blackout.
The reliability of electric power plants is getting higher and higher due to the advancement of technology. Accordingly, some citizens living in metropolitan areas have almost forgotten the risk of blackout. When hundreds of elevators suddenly stop with thousands of passengers trapped within a district, both maintenance contractors and firemen cannot attend all the sites to rescue the passengers on time.
Solutions
Fortunately, the probability of a lightning strike directly hitting a phase wire of an overhead transmission line and causing a voltage sag is not high. Moreover, based on our survey in Hong Kong, most modern elevator controllers can self reset after tripping due to a voltage sag. Also, our test found that under a less serious voltage sag (say, with a voltage of at least 0.8 pu), most elevator controllers and drives are unaffected. While voltage sags due to lightning strikes and blackouts cannot be prevented from happening, something has to be done to mitigate their impact for the sake of passenger safety, health and comfort.
A ride-through device could be installed to tackle the sudden stoppage of the elevator car during a short voltage sag of up to 0.2 s. One such device is a DC backup type able to maintain a more-or-less stable DC bus inside the drive during a voltage sag by temporarily boosting the supply voltage and transferring power from a built-in capacitor inside the device. However, this solution is only applicable to drives with a DC bus, such as a pulse-width-modulated rectifier inverter drive.
Ride-through devices have been installed in escalators in Hong Kong since 2005. According to the region’s Code of Practice on the Design and Construction of Lifts and Escalators Addendum to Amendment No. 8 of 2000 Edition, the braking system of an escalator automatically starts to operate at a lapse of 0.2 s. of a continuous supply voltage sag of more than 10% of the supply voltage, or at a voltage sag exceeding 60% of the supply voltage (without any delay), if the escalator is equipped with such a device to sustain operation during power-supply voltage sags. A sudden stoppage of an escalator could result in the injury of tens of passengers riding on it, which explains why the authority in Hong Kong allows such installation on escalators. Having said that, the authority has had huge concerns about the use of such a device on elevators. The concerns are due to the lack of international standards and product choices in the industry. A delay of braking in 0.2 s. seems to be another concern. Is such a delay that critical?
Whenever something abnormal happens during the operation of an elevator, the brake must be applied on time. Traditionally, the brake is applied by means of a spring when the DC power is removed from the solenoid. Recently, active brake control has been introduced to both elevators and escalators by various researchers and manufacturers.[1 & 2] By using active or intelligent braking, the deceleration rate is under moderate control so that no slipping between the sheave and hoisting ropes is guaranteed. Moreover, if the conventional mechanical brake is used only as a parking brake here, lower wear rates can be sustained. This technology has been applied to automobiles and trains for decades. According to EN 81-1, deceleration under emergency braking must be 0.2-1 g. It is assumed here that the rated speed of an elevator is 5 mps and the deceleration rate is kept constant at 0.2 g (1.96 mps2). The time needed to bring the car to a stop is given by speed/deceleration = 2.55 s. The delay due to the intervention of the ride-through device only accounts for 8% of the whole action.
Besides the impact on the drive, the controller is also very sensitive to voltage sags. Though it is impractical to install an uninterruptible power supply (UPS) to the whole elevator system, with a view to tens of kilowatts, it is not an unreasonable investment to only back up the electronic controller cards by using a UPS to ensure no tripping due to a voltage sag (and, hence, no resetting) is necessary.
Once the elevator suddenly stops (irrespective of either a voltage sag or blackout), the most important strategy is to bring all trapped passengers to the nearest safe landing to let them leave the car. This could be done by the installation of an emergency rescuing device. This device temporarily takes over the controller, drive and brake of the elevator system once tripping occurs. It then checks which side is heavier (i.e., the car side or counterweight side). If the car side is heavier, the device lowers the car to the nearest landing, opens the car/landing doors, closes them afterward and parks the car at this landing until the maintenance technicians arrive onsite to reset everything. A similar set of operational procedures exists if the car side is lighter, but the car is then raised up to the closest safe landing. In either case, a built-in battery ensures the emergency rescuing device works normally long enough to rescue all trapped passengers. From the authors’ point of view, emergency rescuing devices are particularly useful in tackling both voltage sags and blackouts. They have been installed on a large scale – for example, all elevators serving public-housing estates in Singapore are equipped with them.
Finally, even if passengers are trapped under such an emergency condition, it is important that their summons for help be made known. In commercial or industrial buildings unmanned during weekends, there have been incidents in which a trapped passenger had been left alone for hours before being rescued. A “remote alarming, monitoring and communication” system may be the last resort by which to effectively rescue trapped passengers. The whole Chapter 14 of CIBSE Guide D: Transportation Systems in Buildings, 2010 Edition discusses the reasons for remote alarms and monitoring, the functions of it, the information available from the system and its interface with the building management system. By this system, trapped passengers are able to make a service center aware of them and even communicate with the center’s personnel.
Conclusion
Some elevators are sensitive to power-quality issues, and they stop operating, thus trapping passengers. Different ways to tackle this problem have been discussed, including ride-through devices, UPSes, emergency rescuing devices and remote alarming, monitoring and communication systems. It is hoped that readers do not forget the risks of power-quality issues and prepare for them before a related emergency occurs.
Learning-Reinforcement Questions
Use the below learning-reinforcement questions to study for the Continuing Education Assessment Exam available online at www.elevatorbooks.com or on p. 103 of this issue.
♦ Upon a district-wide blackout, how can trapped passengers be rescued when hundreds of elevators fail to operate?
♦ Which devices are sensitive to electric power-supply variations?
♦ What are power quality, power-quality compatibility and electromagnetic compatibility?
♦ What is meant by an active or nonlinear electronic device?
♦ What are “conducted low-frequency phenomena”?
♦ What is the major difference between undervoltage and a voltage sag?
♦ Which component within an elevator system is believed to be most sensitive to voltage sags?
♦ What is the principle of operation of a ride-through device?
References
[1] K. Seaborne, L. Al-Sharif and D. Austin. “Electrically Based Intelligent Escalator Braking Systems,” ELEVATOR WORLD, November 2010, p. 98-108.
[2] R. Kondo, H. Kigawa, T. Ueda, M. Shibata, J. Hashimoto, A. Chida and H. Marumo. “Development of Brake Control System for Elevators,” ELEVATOR WORLD, January 2011, p. 66-72.
Get more of Elevator World. Sign up for our free e-newsletter.