Using a Vector Network Analyzer to Solve Difficult Elevator Problems
Feb 1, 2021
Device can help uncover incompatibilities in elevator equipment that may be causing chronic motion-controller outages.
Early 19th-century elevators, which made multistory buildings feasible, predated electrical distribution systems. These lifts were steam-powered and widely used in industrial facilities. They required steam engineers, lift operators and coal stokers, not to mention the need for cleaning and disposing of ashes. The DC motor in the context of the traction elevator was a vast improvement. With a few simple electrical controls, the motor’s rotation could be reversed, and its speed regulated, which was not possible in an AC motor prior to the introduction of the variable-frequency drive (VFD) in the 1960s.
Gradually, throughout the 20th century and beyond, elevator systems, like automobiles, became more complex, with many improvements making for increased safety and efficiency. First came automatic controls, replacing the human elevator operator. Then, in the early 1980s, the VFD could control an AC induction motor’s speed, direction and torque. These motors were longer-lasting, required less maintenance and caused less electromagnetic interference due to their brushless nature. Around the same time, the elevator motion controller evolved into its modern form: a digital, fully programmable computer linked to the building’s central fire alarm and sprinkler systems with the ability (through two self-testing redundant telephone lines) to automatically call the nearest fire station or other specified location if smoke were detected in the hoistway, machine room or nearby.
If the elevator system detected a problem with the car door, or if one of the hallway doors did not latch securely, power to the motor would be interrupted, and the brake would be applied. All this made for greatly enhanced safety and reliability, overseen by an intricate system of electrical sensors, controls and actuators. The bottom line is that when something went wrong, the computerized motion controller shut down. Often, service could be restored merely by resetting the controller, but this is not always the preferred solution, because the same problem could recur. It is best to find out what went wrong before resetting the motion controller, though this is not always feasible. For example, when a jet crashes, the black box may be destroyed; similarly, data that might indicate a path forward may not survive the motion controller outage.
A reasonable approach, then, involves, for a start, visually examining wiring and components. You can open the motion controller cabinet and check for charred resistors, melted capacitors, loose terminations, etc. Sometimes, this will isolate the fault. However, if a component has overheated to the point of failure, a question remains: “Was the fault intrinsic to the component, or was excessive voltage or loading applied due to an external fault?” Also, don’t neglect outside causes such as ambient heat, vibration or excessive demands on the system.
This sort of examination may yield quick results, but, more often, it leads nowhere, and a more focused approach is needed. Use of electronic instrumentation is usually in order. This can range from a simple neon test light or multimeter, oscilloscope, spectrum analyzer and beyond, including the vector network analyzer (VNA).
According to Rohde & Schwarz’s website (rohde-schwarz. com), the VNA is one of the most essential radio frequency (RF) and microwave approaches. The company offers a wide range of versatile, high-performance network analyzers up to 500 GHz with a maximum of 48 ports. These instruments are suitable for analyzing passive and active components such as filters, amplifiers, mixers and multiport modules.
Tektronix TTR500 VNA permits the user to take precision measurements of all elevator systems, transmission lines and individual components. It is designed to measure reflection coefficients, impedance, admittance, return loss, insertion loss, gain or isolation. The instrument is also suitable for filter measurement, antenna matching and tuning, amplifier measurements and RF cable and connector measurements, among many others.
Keysight (formerly Agilent and, before that, Hewlett-Packard) manufactures network analyzers up to 120 GHz, which can be extended to 1.5 THz with frequency extenders. Additionally, they offer a handheld VNA up to 50 GHz. Its most advanced integrated VNA operates from 10 MHz to 70 GHz (extendable to 1.5 THz). It incorporates three Data Distribution Service sources with low spurious emissions and very low phase noise. Switchable rear-panel jumpers enable signal-conditioning hardware or route additional test equipment to the device under test without the need to move cables.
The vector network analyzer is a costly instrument — more expensive than a high-end digital storage oscilloscope or spectrum analyzer. However, Picotech offers a PC-based VNA, which consists of a rugged module that connects via USB cord to the user’s PC. With the company’s software, the instrument makes use of the PC’s prodigious processing and display capabilities to characterize in-circuit devices, components and transmission lines. This is useful for uncovering incompatibilities in elevator equipment that may be causing chronic motion-controller outages.
Calibration
Calibrating the device differs from the more comprehensive factory calibration, performed on sensitive instruments such as the oscilloscope, spectrum analyzer and VNA. Factory calibrations are typically done before the initial purchase and subsequently once a year or as required. They involve sending the instrument back to the manufacturer, where technicians open the enclosure and do whatever it takes to certify the equipment conforms to original specifications.
The VNA field calibration, in contrast, is performed by the user prior to each new set of measurements. It is performed entirely at a software level without opening the enclosure and takes only a few minutes. It is often performed several times a day (whenever accurate measurements are required). The manual provides complete instructions.
Following installing the software on a PC, electrical connections must be completed. If accurate measurements are required, both the PC and VNA module should be warmed up for about 30 min.
Both Pico VNA models (6 and 8.5 GHz) connect via USB cable to the user-supplied PC. To perform calibration, cables supplied by the manufacturer are run from Port 1 and Port 2 in the module to the device under test. Pico supplies two types of connectors, called “kits,” that attach to the cables. If the insertable device under test has one or two male connectors, use a female connector with a male calibration kit. If the device under test has two male connectors, use female cables and identical male kits for both ports.
Then, open the Pico VNA software. In the main menu on the PC screen, select “Tools and Calibration Kit.” Click “Port 1 Kit,” insert the data for it and click “Apply.” Similarly, click “Load Port 2 Kit,” select the data for it and click “Apply.” The next step is to choose calibration kit(s) appropriate for the device under test. For example, if testing a non-insertable device with female connectors, use a single female kit for both ports in the VNA module.
The next phase in the field calibration procedure is setting up calibration parameters. In the main window, click “Calibration.” Then, perform the following steps:
- Set the sweep parameters. This is like setting up a spectrum analyzer.
- Apply the values.
- Select the measurement required.
- Perform the calibration steps.
- Click Apply Calibration.
The bandwidth setting used during calibration largely determines the available dynamic range during the measurement. For fastest speed, choose 10 kHz. Set calibration power to +0 dBm. Set bandwidth to 140 kHz. For best accuracy and about 100 dB in dynamic range, set calibration bandwidth to 100 Hz. Set calibration power to -3 dBm. Leave bandwidth set to 100 kHz during measurement.
For general use, fast speed, about 90-dB dynamic range, set calibration bandwidth to 1 kHz. Set calibration power to 0 dBm. Leave bandwidth set to 10 Hz during measurement. For best dynamic range, set calibration bandwidth to 10 Hz. Set calibration power to +6 dBm. Leave bandwidth set to 10 Hz during measurement.
In all cases, calibration averaging should be set to “None.” The VNA is not directly concerned with computer networks. The term predates computers. The instrument was used in advanced electronics laboratories when computers were vacuum-tube machines that occupied rows of floor-to-ceiling enclosures. At the time, “networks” were understood to mean electrical networks: generally, devices, components and transmission lines.
The Modern VNA
The VNA, as used today, is a general-purpose instrument that characterizes both linear and nonlinear devices and components, including such diverse types as filters, bridges, attenuators, cables, waveguides, antennae, diodes, transceivers, oscillators, amplifiers and transistors. Unlike many electronic instruments, the VNA does not just measure the device parameters. Instead, it supplies a signal, usually at high frequency, through a cable to the input of the device under test (DUT). It then looks for and times any reflection back through the same cable, much in the manner as radar or a time-domain reflectometer. A time-domain reflectometer is an electronic instrument used to determine the characteristics of electrical lines by observing reflected waveforms. It can be used to characterize and locate faults in metallic cables (for example, twisted pair wire or coaxial cable) or to locate discontinuities in a connector, printed circuit board or any other electrical path.
The VNA also connects to the output of the DUT and characterizes any response it receives. Moreover, by throwing a switch, the user can have the instrument apply the stimulus to the DUT’s output, time and characterize reflections, and monitor the input.
Early VNAs made use of one or more separate, external AFGs, but increasing miniaturization has enabled manufacturers to include everything in a single enclosure. Above all, the VNA performs highly accurate measurements of the ratios of reflected-to-total signal and transmitted-to-total signal.
There are two distinct types of network analyzers. The scalar network analyzer (SNA) measures and characterizes amplitude only, while the VNA measures and characterizes amplitude and phase. Currently, VNAs are far more common than SNAs, because, in today’s high-frequency electronics, phase shift is more likely to occur. It may be intentional (implemented to accomplish a definite purpose) or unintentional and requiring mitigation. In either event, the idea is to detect, measure and precisely categorize phase shift.
Learning-Reinforcement Questions
Use the below learning-reinforcement questions to study for the Continuing Education Assessment Exam available online at www.elevatorbooks.com or on p. 108 of this issue.
- How are the Pico VNA and PC connected?
- Which devices can the VNA characterize?
- How does the VNA supply signals to the DUT?
- What is the difference between a VNA and an SNA?
- How can VNA components be mapped without a schematic diagram?
High frequency (above the utility-supplied 60 Hz) and phase shift play roles in elevator electronics in two ways that are important to the repair technician and design engineer. These have to do with the following:
- The control circuit for an AC elevator drive motor powered by a VFD
- The ubiquitous Controller Area Network (CAN bus).
The VFD is a separate device that, in response to commands from an elevator motion controller, permits a standard three-phase induction motor to run at higher or lower than rated speed and to reverse direction. It also varies the motor’s torque and reports operating status, including temperature and other parameters, back to the motion controller. All this is done over a single Ethernet or other low-voltage circuit distinct from the main power line. This control line runs from the motion controller to the VFD, which is an autonomous unit that may or may not be located within the motion controller cabinet. If an electrical schematic diagram is not available, one can begin mapping out the component parts by following the heavy power lines from the disconnect in the machine room, through the VFD and to the three-phase drive motor.
Unlike many electronic instruments, the VNA does not just measure the device parameters. Instead, it supplies a signal, usually at high frequency, through a cable to the input of the device under test.
CAN bus is a serial bus that was covered in your author’s previous article “CAN Bus for Elevators” (ELEVATOR WORLD, June 2017). It was developed beginning in 1983 by Bosch, a large international manufacturer of electronic systems and components and originally intended for automotive applications, but following its implementation in the 1991 Mercedes-Benz W140, it became widely used in trucks, construction equipment, aircraft, ships and elevators. CAN bus serial data transmission is highly reliable, cost-efficient and tolerant of electrical noise from outside sources. In the latest elevator technology, CAN bus enables serial communication among the many sensors distributed throughout an elevator installation, terminating at the motion controller.
All sensors and actuators, known as nodes, are connected to the motion controller by means of twisted-pair conductors having 120-ohm characteristic impedance. CAN high and CAN low signals are either driven to a dominant state or pulled by resistors to a recessive state. The dominant state is encoded by logic 0 and the recessive state by logic 1. Nodes with lower identifier numbers (IDs) have priority on the bus.
At low frequencies, the wavelength of the signal conveyed through the conductors is invariably far greater than the circuit length. Thus, conventional copper or aluminum conductors are adequate for carrying the power. The wire is sized to have sufficient ampacity so that current and voltage do not vary between transmitter and receiver.
At high frequencies, the signal wavelengths are equal to or smaller than the conductor length. In this situation, the conductors are no longer a pair of wires; instead, they become a transmission line. The material separating the wires is not merely electrical insulation, but a dielectric layer, as in a capacitor. Rather than a flow of electrons, the signal of interest now consists of a succession of traveling waves. This calls for entirely different measuring instrumentation.
At high frequencies, characterization of networks including devices, components and transmission lines requires measurement of phase and magnitude. Measurement of amplitude, as performed by an SNA, is not adequate.
Phase distortion displayed in the VNA reveals group delay, a measure of the transit time of a signal through the DUT, juxtaposed with respect to frequency. It is calculated by comparing the phase of the signal at the input to that at the output. Any variation in group delay gives rise to distortion and is likely to show up as poor performance of a given component or circuit. Deviation from linear phase and group delay are measured and displayed in VNA analysis. Group delay is an easy way to detect the presence of and quantify phase distortion.
An important capability of the VNA is to characterize high-frequency networks. Simple voltage and current measurements at the device ports do not suffice. This is because of probe impedance, among other reasons. Also, active devices may oscillate or be destroyed when shorts and opens are connected to them.
A solution was found in the development of scattering parameters (S-parameters). This refers to gain, loss and reflection as present in traveling waves. These can be readily measured. It is not necessary to compromise an active DUT by exposing it to a harmful load. Moreover, the S-parameters of multiple devices can be tested in cascade. If desired, other parameters can be derived from the S-parameters. These include:
- Admittance parameters (Y-parameters) for linear electrical networks
- Hybrid parameters (h-parameters) for use with a current amplifier
- Impedance parameters (Z-parameters) to describe the electrical behavior of linear electrical networks
The VNA uses four sections to measure the incident, reflected and transmitted signals. They are the source for stimuli, signal-separation devices, receivers that down-convert and detect the signals, and processor and display for interpreting the output.
The source supplies the stimulus for the system being tested. This often consists of a swept frequency. Modern VNAs have internal sources that are synthesized within the instrument. Signal separation is accomplished in the test set. This block performs two functions:
- Measuring part of the incident signal for reference
- Separating the incident and reflected traveling waves at the DUT input
The signal detection block may consist of a diode detector that converts the RF signal level to a proportional DC level. Diode detectors, cheaper than tuned receivers, provide broadband response. Their broadband response, however, limits sensitivity and makes them prone to spurious signals including harmonics. More accurate measurements are obtained by AC detection.
Tuned receivers use heterodyne technology, mixing the RF down to a more user-friendly intermediate frequency (IF). The local oscillator is locked to the RF or IF signal so the receivers are consistently tuned to the RF signal. Analog-to-digital conversion and digital processing extract magnitude and phase information from the IF signal.
The VNA’s display-processor section is where reflection and transmission data are presented to the user. Prominent features are markers; limit lines; pass/fail indicators; linear and log formats; and grid, polar and Smith charts. Most of this is self-explanatory, but we will discuss the widely used Smith chart, which figures prominently in the VNA display. The Smith chart is a polar graphical calculator that enables RF engineers to solve transmission-line and matching circuit problems.
The chart, devised by Phillip H. Smith (1905-1987) and T. Mizuhashi, is used to display electronic parameters such as impedances, admittances, reflection coefficients, S-parameters, noise figure circles, constant gain contours and regions of unconditional stability. Today, software-based methods have replaced the physical chart to a great extent, but it still appears in the VNA display and is useful in organizing information provided by the instrument. Reflection coefficients can be read directly from the chart.
Around its circumference is a scale graduated in wavelengths and degrees. It represents the distance along a transmission line between source and load. The Smith chart uses the standard reflection coefficient formula to develop equations for circles with various radii. The Smith chart is an array of circles, each located in a different place relative to the plot and each representing constant resistance or constant reactance, the parameters that comprise constant impedance.
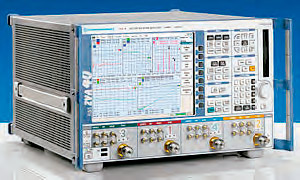
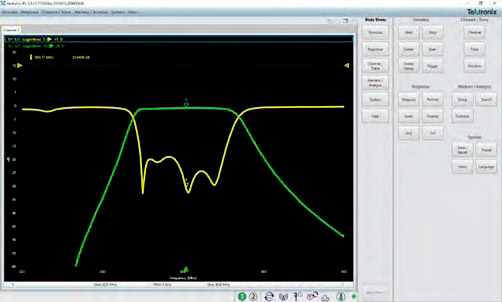
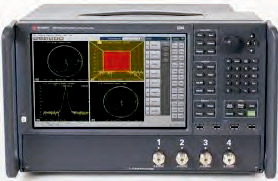
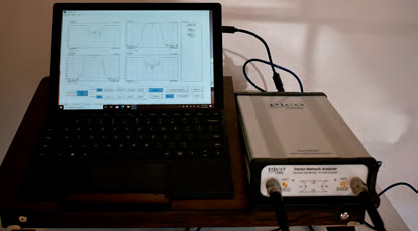
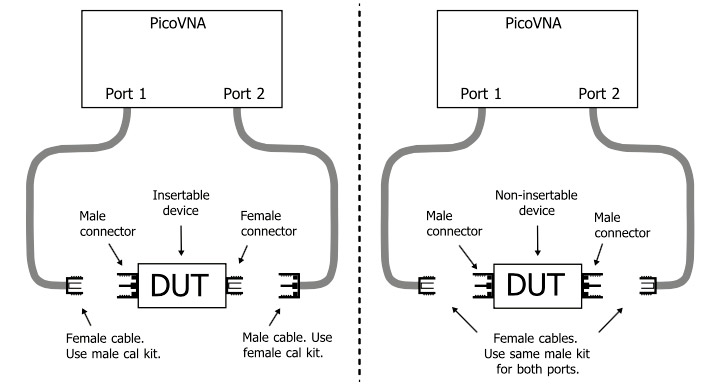
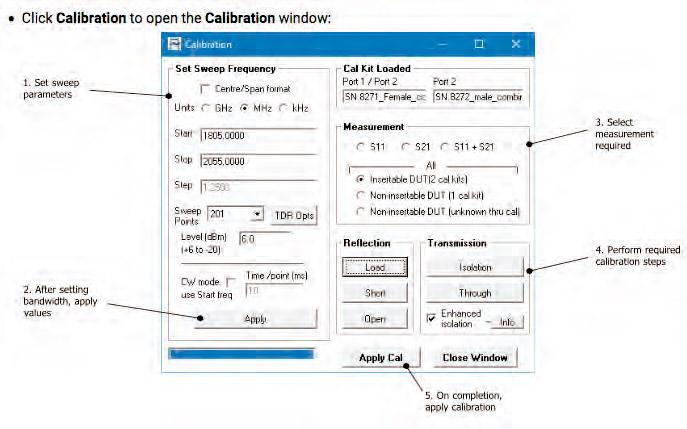
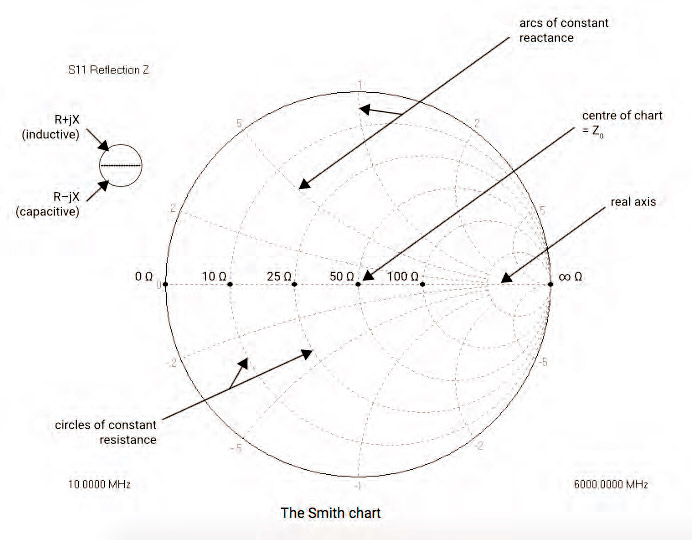
Get more of Elevator World. Sign up for our free e-newsletter.